The main feature that distinguishes electronic circuits from the circuits we have learned before is the introduction of various electronic devices into the circuits. There are many types of electronic devices, and the most widely used ones are semiconductor devices - diodes, voltage regulators, transistors, insulated gate field effect transistors, etc. Since the task of this course is not to study the physical processes inside these devices, but to discuss their applications, therefore, based on a brief introduction to the external characteristics of these devices, their application circuits are discussed.
4.1 PN Junction and Semiconductor Diode
4.1.1 Unidirectional conductivity of PN junction
We have learned in physics that when a trace amount of trivalent (such as boron) or pentavalent (such as phosphorus) elements is doped into a pure tetravalent semiconductor crystal material (mainly silicon and germanium), the conductivity of the semiconductor will be greatly enhanced. This is due to the formation of carriers capable of conducting current. The majority carriers in a semiconductor doped with pentavalent elements are free electrons, which are called electron semiconductors or N-type semiconductors. The majority carriers in a semiconductor doped with trivalent elements are holes, which are called hole semiconductors or P-type semiconductors. In a doped semiconductor, the number of majority carriers (called majority carriers) is determined by the doping concentration, while the number of minority carriers (called minority carriers) is related to temperature, and the number of minority carriers increases when the temperature rises.
On a semiconductor substrate, the junction of a P-type semiconductor and an N-type semiconductor can be formed through appropriate semiconductor process technology, which is called a PN junction. The PN junction has unidirectional conductivity: when a forward voltage is applied to the PN junction, the potential of the P terminal is higher than that of the N terminal, the PN junction becomes narrower, and the current formed by the majority carriers can flow from the P region to the N region, as shown in Figure 4-1 (a). When a reverse voltage is applied to the PN junction, the potential of the N terminal is higher than that of the P terminal, the PN junction becomes wider, and the current formed by the minority carriers is extremely small, which is considered to be cut off (non-conducting), as shown in Figure 4-1 (b).
4.1.2 Semiconductor diode
A semiconductor diode is made up of a PN junction plus corresponding electrode leads and tube shell packaging. The electrode leading out of the P region is called the anode, and the electrode leading out of the N region is called the cathode. Because of the unidirectional conductivity of the PN junction, when the diode is turned on, the current flows from the anode through the inside of the tube to the cathode. There are many types of diodes. According to the material, the most commonly used are silicon tubes and germanium tubes; according to the structure, there are point contact type, surface contact type and silicon planar type; according to the purpose, there are ordinary diodes, rectifier diodes, voltage regulator diodes and many other types.
Figure 4-2 is a schematic diagram of the symbol, structure and appearance of commonly used diodes. The symbol of the diode is shown in Figure 4-2 (a). The arrow indicates the direction of the forward current. Generally, this symbol or a color dot or color circle is marked on the surface of the diode shell to indicate the polarity of the diode. The solid arrow symbol on the left is a commonly used symbol in engineering, and the symbol on the right is a newly specified symbol. From the perspective of process structure, the point contact diode (usually a germanium tube) is shown in Figure 4-2 (b). Its characteristics are small junction area, small junction capacitance, and small current allowed to pass. It is suitable for detection of high-frequency circuits or rectification of small currents, and can also be used as a switching element in digital circuits; the surface contact diode (usually a silicon tube) is shown in Figure 4-2 (c). Its characteristics are large junction area, large junction capacitance, and large current allowed to pass. It is suitable for low-frequency rectification; the silicon planar diode is shown in Figure 4-2 (d). The large junction area can be used for high-power rectification, and the small junction area is suitable for pulse digital circuits as a switching tube. 4.1.3 The volt-ampere characteristics of the diode
The relationship curve between the current and voltage of the diode is I = f (V), which is called the diode's volt-ampere characteristic. Its volt-ampere characteristic curve is shown in Figure 4-3. The core of the diode is a PN junction with unidirectional conductivity. Its actual volt-ampere characteristic is slightly different from the theoretical volt-ampere characteristic. As shown in Figure 4-3, the diode's volt-ampere characteristic curve is nonlinear and can be divided into three parts: forward characteristic, reverse characteristic and reverse breakdown characteristic.
1. Forward characteristics
When the external forward voltage is very low, the diffusion movement of most carriers in the tube is not formed, so the forward current is almost zero. When the forward voltage exceeds a certain value, there is a significant forward current. This voltage value is called the dead zone voltage. Usually, the dead zone voltage of silicon tubes is about 0.5V, and the dead zone voltage of germanium tubes is about 0.2V. When the forward voltage is greater than the dead zone voltage, the forward current increases rapidly, and the curve is close to the rising straight line. In this part of the volt-ampere characteristic, when the current increases rapidly, the forward voltage drop of the diode changes very little. The forward voltage drop of silicon tubes is about 0.6~0.7V, and the forward voltage drop of germanium tubes is about 0.2~0.3V. The volt-ampere characteristic of the diode is very sensitive to temperature. When the temperature rises, the forward characteristic curve shifts to the left, as shown in Figure 4-3, which shows that for the same forward current, the forward voltage drop decreases with the temperature rise. Studies have shown that the forward voltage drop decreases by 2mV for every 10C increase in temperature.
2. Reverse characteristics
When a reverse voltage is applied to a diode, a very small reverse current is formed, and its amount remains basically unchanged at a certain temperature. Therefore, when the reverse voltage increases within a certain range, the magnitude of the reverse current is basically constant, and has nothing to do with the magnitude of the reverse voltage. Therefore, it is called the reverse saturation current. Generally, the reverse current of a low-power germanium tube can reach tens of μA, while the reverse current of a low-power silicon tube is much smaller, generally below 0.1 μA. When the temperature rises, the number of minority carriers increases, which increases the reverse current and shifts the characteristic curve downward. Studies have shown that the reverse current approximately doubles for every 100°C increase in temperature.
3. Reverse breakdown characteristics
When the external reverse voltage of a diode is greater than a certain value (reverse breakdown voltage), the reverse current suddenly increases sharply, which is called diode reverse breakdown. The reverse breakdown voltage is generally more than tens of volts.
4.1.4 Main parameters of diode
In addition to the volt-ampere characteristic curve, the diode's parameters can also reflect the diode's electrical performance. The device parameters are the basis for the correct selection and use of the device. The parameters of various devices are given in the manufacturer's product manual. Due to manufacturing process reasons, even the same type of tube has a certain dispersion of parameters, so the manual often gives a range of certain parameters. The main parameters of semiconductor diodes are as follows: 1. Maximum rectified current IDM
IDM refers to the maximum average forward current allowed to pass through the diode when it is working for a long time. When in use, if the current exceeds this value, the PN junction will overheat and burn out the tube.
2. Reverse working peak voltage VRM
VRM refers to the maximum reverse voltage allowed for the tube to not break down. Generally, this parameter is half of the reverse breakdown voltage of the diode. If the reverse voltage exceeds this value, the tube will be in danger of breaking down.
3. Reverse peak current IRM
IRM refers to the reverse current value when the reverse voltage VRM is applied to the diode. The smaller the IRM, the better the unidirectional conductivity of the diode. IRM is greatly affected by temperature, so be careful when using it. The reverse current of silicon tubes is small, generally below a few microamperes, while the reverse current of germanium tubes is large, which is dozens to hundreds of times that of silicon tubes.
4. Maximum operating frequency ƒM
When a high-frequency AC voltage is applied to a diode, the unidirectional conductive function degrades due to the capacitance effect of the PN junction. ƒM refers to the frequency of the AC signal at which the unidirectional conductive function of the diode begins to degrade significantly.
4.1.5 Diode Equivalent Circuit and Its Application
Since the volt-ampere characteristic of a diode is nonlinear, for the convenience of analysis and calculation, under certain conditions, we can linearize it and regard it as an ideal component.
1. Ideal diode equivalent circuit
In a circuit, if the forward voltage drop of a diode when it is turned on is much smaller than the voltage of the element in series with it, and the reverse current of a diode when it is turned off is much smaller than the current of the element in parallel with it, then the forward voltage drop and reverse current of the tube can be ignored and the diode can be idealized as a switch. When a forward voltage is applied, the diode is turned on and the forward voltage drop is 0, which is equivalent to a closed switch. When a reverse voltage is applied, the diode is turned off and the reverse current is 0, which is equivalent to an open switch. The equivalent circuit of an ideal diode is shown in Figure 4-4. Using an ideal diode to represent an actual diode for circuit analysis and calculation can produce relatively satisfactory results, but there are some errors.
2. Examples of diode application circuits
The diode has a wide range of applications, mainly using its unidirectional conductivity. Here are some application circuits.
(1) Limiting circuit: The function of the limiter is to limit the amplitude of the output voltage.
Example 4-1 Figure 4-5 (a) is a circuit diagram that uses a diode as a forward limiter. Given vi = Vmsinωt, and Vm>VS, analyze the working principle and plot the waveform of the output voltage vo.
Solution: a) The condition for the diode to conduct is vi>VS. Since D is an ideal diode, once D is conducted, the tube voltage drop is zero. At this time, vo=VS
b) When vi≤VS, the diode is cut off, the branch is disconnected, there is no current in R, and its voltage drop is 0. So vo = vi
c) Based on the above analysis, the waveform of vo can be drawn, as shown in Figure 4-5 (b). It can be seen from the figure that the positive amplitude of the output voltage is limited to the VS value.
Note: When drawing the graph, the waveforms of vo and vi must correspond on the time axis, so as to correctly reflect the changing process of vo.
(2) Diode gate circuit
A gate circuit is a logic circuit in which there is a certain cause-and-effect relationship, i.e., a logical relationship, between the input signal (condition) and the output signal (result). In a logic circuit, the symbols 0 and 1 are usually used to represent two opposing logical states. 1 represents a high level, and 0 represents a low level, which is called positive logic, and vice versa, negative logic. There are three basic logical relationships: AND logic, OR logic, and NOT logic. The corresponding gate circuits are AND gate, OR gate, and NOT gate. These three basic gate circuits can form a variety of other composite gate circuits.
Example 4-2 Figure 4-6 shows the simplest AND gate circuit and logic diagram symbol. It is composed of diodes D1, D2, resistor R and power supply VCC. In the figure, A and B are two input terminals, and F is the output terminal. Assume VCC = 5V, the high level (logic 1) of the A and B input terminals is 3V, the low level (logic 0) is 0V, and the forward voltage drop of diodes D1 and D2 is ignored. Try to analyze the relationship between the input and output of the circuit.
Solution: (1) When the input terminals A and B are both at low level 0, that is, VA = VB = 0V, diodes D1 and D2 are forward biased and turned on, making the voltage VF at the output terminal F = 0V, that is, the output terminal F is at low level 0.
(2) When input terminal A is at a low level 0 and input terminal B is at a high level 1, that is, VA = 0 V and VB = 3 V, the cathode potential of D1 is lower than the cathode potential of D2, and D1 is turned on, making VF = 0 V. Therefore, D2 is reverse biased and cut off, and the output terminal F is at a low level 0.
(3) When input terminal A is at high level 1 and input terminal B is at low level 0, that is, VA = 3V, VB = 0V, the working conditions of D1 and D2 are opposite to (2), and the output terminal F is still at low level 0.
(4) When the input terminals A and B are both at high level 1, that is, VA = VB = 3V, D1 and D2 are both forward biased and turned on, making the voltage VF at the output terminal F = 3V, that is, the output terminal F is at high level 1.
From the above analysis, we can know that only when all input terminals are high level 1, the output terminal is high level 1, otherwise the output terminals are all low level 0. This kind of "only when all conditions that determine the result of an event are met, the result occurs" logical relationship is called AND logic, and the AND gate circuit satisfies the AND logic relationship. AND logic is also called logical multiplication and AND operation. It is usually represented by the symbol "·". If A, B, and F are logical variables, the expression of AND operation can be written in the following form:
F=A·B or F=AB
The above formula is read as F equals A and B. The meaning of logical AND is: only when the input variables A and B are both 1, the output variable F is 1; as long as one of A and B is 0, F is 0. In other words, "0 is output if there is 0, and 1 is output if all are 1". This conclusion is also applicable to AND operations involving multiple variables.
Table 4-1 lists the relationship between the input and output logic levels of the circuit shown in Figure 4-6. However, in logic circuit analysis, logic 0 and 1 are usually used to describe the relationship between input and output. The table listed is called a truth table (i.e., a logic state table). The truth table of the AND gate above is shown in Table 4-2.
In addition, Figure 4-7 shows the OR gate circuit and logic diagram symbol. It is also composed of diodes and resistors. In the figure, A and B are two input terminals, and F is the output terminal. Assume that the high level (logic 1) of the A and B input terminals is 3V, and the low level (logic 0) is 0V, and ignore the forward voltage drop of diodes D1 and D2. Through analysis (readers can analyze the detailed process by themselves), it can be seen that as long as one of A and B is high level (logic 1), the output is high level (logic 1). Only when A and B are low level (logic 0) at the same time, the output is low level (logic 0). This kind of "among all the conditions that determine the result of an event, the result will occur as long as one or more of them are met" logical relationship is called OR logic. The OR gate circuit satisfies the OR logic relationship.
OR logic is also called logical addition or OR operation. It is usually represented by the symbol "+". If A, B, and F are logical variables, the expression of OR operation can be written in the following form:
The formula F=A+B is read as F equals A or B. The meaning of logical OR is: only when one or more of the input variables A and B is 1, the output variable F is 1; conversely, only when A and B are all 0, F is 0. In other words, "if there is 1, the output is 1, and if all are 0, the output is 0." This conclusion also applies to OR operations involving multiple variables.
Table 4-3 lists the relationship between the input and output logic levels of the circuit shown in Figure 4-7. Table 4-4 is the truth table of the above OR gate.
In addition to the common diodes mentioned above, there are some special diodes, such as Zener diodes, photodiodes, light-emitting diodes, etc., which are introduced as follows.
4.2.1 Zener diode
The Zener diode is a special surface-contact semiconductor silicon diode that has the function of stabilizing voltage. Figure 4-8 (a) shows the correct connection method of the Zener diode in the circuit; Figures (b) and (c) show the volt-ampere characteristics and graphic symbols of the Zener diode. The main difference between the Zener diode and the ordinary diode is that the Zener diode works in the reverse breakdown state of the PN junction. By taking process measures in the manufacturing process and limiting the size of the reverse current during use, it can be ensured that the Zener diode will not be damaged due to overheating in the reverse breakdown state. It can be seen from the reverse characteristic curve of the Zener diode that when the reverse voltage is small, the reverse current is almost zero. When the reverse voltage increases to the breakdown voltage Vz (which is also the working voltage of the Zener diode), the reverse current Iz (the working current of the Zener diode) will increase sharply, and the Zener diode will reversely break down. In the ab section of the characteristic curve, when Iz changes within a large range, the voltage Vz across the Zener diode remains basically unchanged, and has a constant voltage characteristic. This characteristic can be used to stabilize the voltage.
The Zener diode is different from the general diode. Its reverse breakdown is reversible. As long as it does not exceed the allowable value of the Zener diode, the PN junction will not be damaged by overheating. When the external reverse voltage is removed, the Zener diode restores its original performance, so the Zener diode has good repeated breakdown characteristics.
The main parameters of the voltage regulator are:
1. Stable voltage VZ. Stable voltage VZ refers to the voltage across the tube when the voltage regulator works normally. Due to the manufacturing process, the voltage value also has a certain dispersion. For example, the voltage value of 2CW14 is 6.0~7.5V.
2. Dynamic resistance rz. Dynamic resistance refers to the ratio of the change in terminal voltage to the change in corresponding current within the normal working range of the voltage regulator.
The steeper the reverse characteristic of the voltage regulator, the smaller the rZ, and the better the voltage regulation performance.
3. Stable current IZ. The reference current value when the voltage regulator is working normally. Only when I≥IZ can the voltage regulator have a good voltage stabilization performance.
4. Maximum stable current IZmax. The maximum reverse current allowed to pass. If I > IZmax, the tube will be damaged due to overheating.
5. Maximum allowable power consumption PZM. The maximum power loss that will not cause thermal breakdown of the tube PZM = VZ IZmax
6. Voltage temperature coefficient αV. When the temperature changes by 10°C, the percentage of stable voltage change is defined as voltage temperature coefficient. The smaller the voltage temperature coefficient, the better the temperature stability. Usually, silicon voltage regulator has a negative temperature coefficient when VZ is lower than 4V and a positive temperature coefficient when it is higher than 6V. When VZ is between 4 and 6V, the temperature coefficient is very small.
There are two conditions for the normal operation of the Zener diode. One is that it works in the reverse breakdown state, and the other is that the current in the Zener diode must be between the stable current and the maximum allowable current. When the Zener diode is forward biased, it is equivalent to an ordinary diode. Figure 4-8 (a) is the most commonly used voltage stabilization circuit. When Vi or RL changes, the current in the Zener diode changes, but within a certain range, its terminal voltage changes very little, so it plays a role in stabilizing the output voltage. (See Section 4.4 for the circuit analysis) 4.2.2 Photodiode
Photodiode is also called photosensitive diode. There is a glass window on its tube shell to receive light. Its characteristic is that when light shines on its PN junction, it can generate free electrons and holes in pairs, which increases the concentration of minority carriers in the semiconductor. These carriers can generate drift current under a certain reverse bias voltage, which increases the reverse current. Therefore, its reverse current increases linearly with the increase of light intensity. At this time, the photodiode is equivalent to a constant current source. When there is no light, the volt-ampere characteristic of the photodiode is the same as that of an ordinary diode. The equivalent circuit of the photodiode is shown in Figure 4-9 (a), and Figure 4-9 (b) is the symbol of the photodiode.
Dark current: Reverse saturation current when there is no light. Generally <1μA.
Photocurrent: refers to the reverse current under rated illumination, generally tens of milliamperes.
Sensitivity: refers to the photocurrent generated by the photodiode at a given wavelength (such as 0.9μm) per unit light power. Generally ≥ 0.5μA/μW.
Peak wavelength: The wavelength of light that gives the photodiode the highest response sensitivity (maximum photocurrent). Generally, the peak wavelength of a photodiode is in the visible and infrared range.
Response time: refers to the time required for the photocurrent to reach 63% of the stable value after adding a certain amount of light, generally 10-7S.
Photodiodes can be used as light control elements for various object detection, photoelectric control, automatic alarm, etc. When large-area photodiodes are made, they can be used as a source of energy and are called photocells. At this time, it does not require an external power supply and can directly convert light energy into electrical energy.
4.2.3 Light Emitting Diodes
A light emitting diode is a semiconductor solid-state display device that converts electrical energy directly into light energy, referred to as LED (Light Emitting Diode). Similar to ordinary diodes, light emitting diodes are also composed of a PN junction. The PN junction of the light emitting diode is encapsulated in a transparent plastic shell, and the shape is square, rectangular, and circular. The light emitting diode has low driving voltage and low operating current. It has strong vibration and impact resistance, small size, high reliability, low power consumption, and long life. It is widely used in signal indication circuits. The digital tube commonly used in electronic technology is composed of light emitting diodes arranged in a certain way.
The principle of light-emitting diodes is opposite to that of photodiodes. When this tube is forward biased and current passes through it, it will emit light. This is the result of the release of energy when electrons and holes are directly recombined. Its spectral range is relatively narrow, and its wavelength is determined by the basic materials used. Light-emitting diodes made of different semiconductor materials emit different colors of light, such as gallium arsenide phosphide (GaAsP) materials emit red or yellow light, gallium phosphide (GaP) materials emit red or green light, gallium nitride (GaN) materials emit blue light, silicon carbide (SiC) materials emit yellow light, and gallium arsenide (GaAs) materials emit invisible infrared light.
The symbol of the light-emitting diode is shown in Figure 4-10. Its volt-ampere characteristics are similar to those of ordinary diodes, with a dead zone voltage of 0.9~1.1V, a forward operating voltage of 1.5~2.5V, and an operating current of 5~15mA. The reverse breakdown voltage is low, generally less than 10V.
4.3 Diode Rectification and Filtering Circuit
In the circuit, a DC power supply with stable voltage is usually required. The composition of a small power stabilized power supply can be shown in Figure 4-11. It consists of four parts: power transformer, rectifier, filter and voltage stabilizer circuit.
The power transformer converts the AC grid voltage into the required voltage value, and then converts the AC voltage into a pulsating DC voltage through a rectifier circuit. Since this pulsating DC voltage also contains large ripples, it must be filtered out through a filter circuit to obtain a smooth DC voltage. However, such a voltage also changes with grid voltage fluctuations (generally with a fluctuation of about ±10%), load and temperature changes. Therefore, after the rectification and filtering circuits, a voltage stabilization circuit is required. The function of the voltage stabilization circuit is to maintain the output DC voltage stable when the grid voltage fluctuates, the load and the temperature change.
4.3.2 Filter Circuit
Although the rectifier circuit converts AC into DC, the output is a pulsating voltage. This pulsating voltage with varying magnitudes contains not only DC components but also AC components of different frequencies, which is far from meeting the power supply requirements of most electronic devices. In order to improve the pulsation of the rectified voltage and improve its smoothness, a filter must be added to the rectifier circuit. The following introduces several commonly used filter circuits.
1. Capacitor filter circuit
The capacitor filter circuit is the simplest filter. It is composed of a capacitor C connected in parallel with the load at the output end of the rectifier circuit, as shown in Figure 4-15 (a).
Capacitor filtering filters out AC components by charging and discharging capacitors. The dotted waveform in the waveform diagram of Figure 4-15 (b) is the waveform of half-wave rectification. After the capacitor C is added, when v2>0, D is turned on. While the power supply is supplying power to RL, it also charges C to store energy. Since the charging time constant is very small (the winding resistance and the forward resistance of the diode are very small), the charging is very fast, and the output voltage vo rises with v2. When vC =, v2 begins to decrease. After v2 In order to achieve the value relationship of formula (4-10) and obtain a relatively flat output voltage, it is generally required Where T is the period of the AC voltage of the power supply. In addition, since the conduction time of the diode is short (the conduction angle is less than 1800), and the average current of the capacitor is zero, it can be seen that the average current when the diode is turned on is equal to the average current of the load. Therefore, the current peak of the diode must be large, resulting in current shock, which can easily damage the tube. The maximum reverse working voltage of the diode in the rectifier circuit with capacitor filtering is not equal for half-wave and full-wave rectifier circuits. In the half-wave rectifier circuit, the most serious situation to be considered is that the output end is open, the capacitor is charged with V2m, and v2 is at the amplitude of the negative half cycle. At this time, the diode is subjected to a reverse working voltage of 2V2. It is doubled compared to the case without filtering capacitors. For a single-phase bridge rectifier circuit, whether there is a filter capacitor or not, the highest reverse operating voltage of the diode is V2. The selection of filter capacitor value should be determined by the load current. Generally, it is between tens of microfarads and thousands of microfarads. The capacitor withstand voltage should be greater than the maximum output voltage. Polar capacitors are usually used.
Previous article:RS allows you to wait no more, a milestone in the evolution of PCB design and manufacturing
Next article:Introducing a simple method of drawing circuit diagrams
Recommended ReadingLatest update time:2024-11-15 19:35
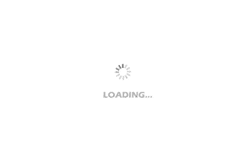
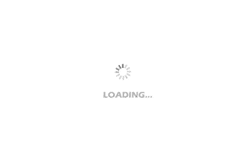
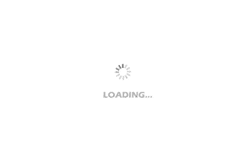
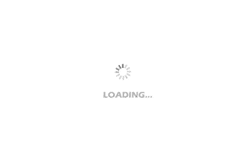
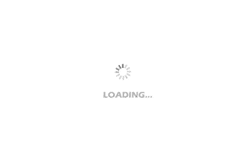
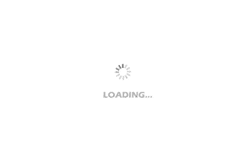
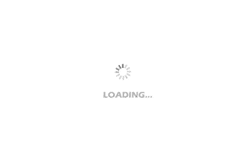
- Popular Resources
- Popular amplifiers
-
Modern Electronic Technology Training Course (Edited by Yao Youfeng)
-
Sensor Principle and Application Circuit Design (Edited by Chen Shuwang, Song Lijun, Xu Yunfeng)
-
Single-chip microcomputer C language programming and simulation
-
100 Examples of Microcontroller C Language Applications (with CD-ROM, 3rd Edition) (Wang Huiliang, Wang Dongfeng, Dong Guanqiang)
- MathWorks and NXP Collaborate to Launch Model-Based Design Toolbox for Battery Management Systems
- STMicroelectronics' advanced galvanically isolated gate driver STGAP3S provides flexible protection for IGBTs and SiC MOSFETs
- New diaphragm-free solid-state lithium battery technology is launched: the distance between the positive and negative electrodes is less than 0.000001 meters
- [“Source” Observe the Autumn Series] Application and testing of the next generation of semiconductor gallium oxide device photodetectors
- 采用自主设计封装,绝缘电阻显著提高!ROHM开发出更高电压xEV系统的SiC肖特基势垒二极管
- Will GaN replace SiC? PI's disruptive 1700V InnoMux2 is here to demonstrate
- From Isolation to the Third and a Half Generation: Understanding Naxinwei's Gate Driver IC in One Article
- The appeal of 48 V technology: importance, benefits and key factors in system-level applications
- Important breakthrough in recycling of used lithium-ion batteries
- LED chemical incompatibility test to see which chemicals LEDs can be used with
- Application of ARM9 hardware coprocessor on WinCE embedded motherboard
- What are the key points for selecting rotor flowmeter?
- LM317 high power charger circuit
- A brief analysis of Embest's application and development of embedded medical devices
- Single-phase RC protection circuit
- stm32 PVD programmable voltage monitor
- Introduction and measurement of edge trigger and level trigger of 51 single chip microcomputer
- Improved design of Linux system software shell protection technology
- What to do if the ABB robot protection device stops
- Car key in the left hand, liveness detection radar in the right hand, UWB is imperative for cars!
- After a decade of rapid development, domestic CIS has entered the market
- Aegis Dagger Battery + Thor EM-i Super Hybrid, Geely New Energy has thrown out two "king bombs"
- A brief discussion on functional safety - fault, error, and failure
- In the smart car 2.0 cycle, these core industry chains are facing major opportunities!
- The United States and Japan are developing new batteries. CATL faces challenges? How should China's new energy battery industry respond?
- Murata launches high-precision 6-axis inertial sensor for automobiles
- Ford patents pre-charge alarm to help save costs and respond to emergencies
- New real-time microcontroller system from Texas Instruments enables smarter processing in automotive and industrial applications
- Sandia Labs develops battery failure early warning technology to detect battery failures faster
- Forum friends give examples to explain these operators in C language
- Talk about the upper and lower tubes of the buck circuit_DC-DC BUCK bootstrap circuit
- Real-time solution to single chip microcomputer delay
- CB5654 Development Board Review 2
- [Raspberry Pi Pico Review] 3. My first project
- Problems with op amp sampling in oximeters
- EasyARM-RT1052 Review Summary
- If you have original works, I will give you rewards, up to 500 yuan in cash rewards
- Submit your idea, experience MPS products or evaluation boards for free, and win a backpack, Bluetooth wireless headset, and 10,000 yuan in cash!
- Power supply obstacles + small mistakes in charging management