By: Bernd Heidtmann, Product Manager, Standard Precision GNSS Product Strategy, u-blox AG
Summary
Satellite positioning is a key enabler for a growing number of consumer, industrial, and automotive tracking applications, due to its global availability and high accuracy. At the same time, users continue to have higher expectations for satellite positioning technology in terms of performance, size, power consumption, and cost. In order to optimally balance these characteristics to meet the needs of a specific application or use case, a deep understanding of the technologies, hardware, software, and services used is required. In this white paper, we provide our insights into various GNSS tracking device power reduction strategies, which reflect our more than 20 years of innovation in this field.
1. Best performance and lowest power consumption
GNSS technology has a place in the emerging smart connected solutions due to its ability to provide highly accurate position, velocity and time data on a global scale. At the same time, users’ expectations of GNSS technology are also increasing. To stand out in today’s competitive market, GNSS devices need to outperform competitors in terms of accuracy, responsiveness and power consumption, which often comes with great pressure to reduce cost and size.
Global Navigation Satellite System (GNSS) receivers have long been considered power hogs, especially in consumer, industrial, and automotive tracking use cases. Back in 2010, single-band receivers consumed a little over 120 mW in continuous tracking mode. By 2015, power consumption had dropped to around 70 mW. Today, thanks to technology improvements, tracking applications consume just 25 mW.
Over the past five years, the power consumption of GNSS technology has reached new heights. Today’s low-power GNSS receivers can track more satellite constellations, each supporting multiple frequency bands, providing higher positioning accuracy at faster speeds and lower power consumption. In some use cases, the power consumption of GNSS receivers can be reduced to less than ten percent of the end device’s power budget.
However, achieving this low power goal is challenging. Currently, advanced GNSS receivers typically offer a range of settings that users can configure to optimize power consumption while meeting the performance requirements of specific use cases.
In this white paper, we outline some basic design considerations that can be used to enable GNSS receivers to consume a fraction of the power budget of standard GNSS solutions. The design factors that need to be considered for a specific use case will depend on the user's trade-offs between accuracy, dynamic performance, size, cost, etc.
Power distribution between cellular communications and GNSS for tracking devices using cloud-based positioning technology.
2. Use case determines power saving options
The power saving options available to product developers to balance the accuracy, performance, size and cost of GNSS receivers depend largely on their application scenarios. For example, sports watches are small in size and usually only have small antennas and batteries, so they require relatively high 1 Hz update rates and have demanding requirements for GNSS performance. Most sports watches only provide limited Internet connectivity (via smartphones).
Logistics cargo trackers have more relaxed requirements in terms of update rate and GNSS receiver performance, but need to run for months on a small battery. In between are handheld devices and pet and child trackers.
Car trackers are in a class of their own, with features including low size constraints, mains power, and constant communication using cellular data.
The following table outlines common limits for five categories of end devices. The specific limits for a given end device will depend on the specific requirements dictated by its use case and may differ from the examples provided below.
Typical limits for five common types of terminal equipment.
3. Performance and power consumption
As we can see, the power consumption of a GNSS receiver is determined by a number of factors. Most of the power is consumed during startup (satellite signal acquisition), which typically consumes around 20% more than continuous satellite tracking, so the main drivers of power consumption are related to satellite signal acquisition and continuous satellite tracking.
In addition to the size and cost of the end device, the performance requirements of the GNSS in the application will also limit the options available to reduce power consumption. As we can see, each of the above options has its own set of trade-offs that developers need to carefully consider to fully meet the needs of the target application.
Availability and accuracy
While some use cases require highly accurate GNSS positioning at all times, other use cases have less stringent requirements. Today’s most advanced GNSS receivers offer a variety of ways to balance the conflicting demands of positioning availability and accuracy versus power consumption.
The first method involves selecting the number of GNSS constellations tracked. Tracking multiple GNSS constellations simultaneously increases the number of satellites visible at any given time, thereby improving the robustness of positioning readings. In environments with poor signal conditions, such as deep in urban canyons or under forest canopies, this approach can significantly improve the availability, accuracy, and reliability of positioning services.
In terms of power consumption, tracking more GNSS constellations forces the receiver to spend more time acquiring satellite signals, which in turn increases power consumption. In addition, when the receiver tracks GNSS signals in different frequency bands, it requires additional RF paths to acquire signals, which also increases power consumption.
The second approach focuses on antenna selection, that is, choosing an antenna that meets the performance requirements of the final application. Active antennas use low-noise amplifiers (LNAs) to increase the gain of the input RF signal, which is critical for capturing weak satellite signals in the noise, especially in weak signal scenarios such as when the antenna is improperly placed or indoors. However, active antenna LNAs consume considerable power to achieve the required antenna gain, and such increased sensitivity usually comes at the expense of increased power consumption. Therefore, for low-power use cases that require excellent positioning availability and accuracy, large passive antennas can be used (with some compromise in the size of the terminal device).
Tracking Mode
While some use cases require continuous tracking, others only need to read positioning data no more than once per minute, hour, or even day. The positioning update rate determines the time interval between two position calculations and can significantly affect the power consumption of the GNSS receiver.
On this graph, continuous tracking mode has the highest power consumption. In applications that combine GNSS positioning and LTE cloud connectivity, continuous tracking mode also introduces the challenge of RF interference between the transmitted LTE signal and the GNSS signal received in a nearby frequency band.
Effective RF interference mitigation can reduce the power consumption of GNSS receivers by preventing signal loss from forcing the receiver to re-enter the power-hungry signal acquisition phase. RF interference mitigation requires an upfront investment in precision board design and may require additional filters, which increase signal attenuation in the RF path. When using a passive antenna setup, it may be necessary to add a low noise amplifier (LNA).
For applications that do not require continuous tracking, many GNSS receivers offer a power saving mode (PSM) that significantly reduces power consumption by limiting the GNSS receiver's tracking capabilities between position calculations.
There are several types of PSM, each with different advantages and disadvantages. To achieve the best balance between receiver performance and power consumption, developers need to wisely choose the PSM that best meets the needs of the use case.
4. Hardware-based power consumption optimization strategy
Designers of tracking solutions can use a full set of design strategies to optimize the power consumption of their devices. Since each decision affects not only the power consumption, but also the performance, size, and cost of the tracking solution, the solution designer must carefully weigh the pros and cons of each strategy to find the lowest power configuration that provides the required tracking performance.
Best Component Selection
Selecting low-power components (LNA, crystal, real-time clock) will slightly improve the overall power consumption of the GNSS receiver during operation.
power supply
Although GNSS devices operate within a narrow voltage range, there is an optimal voltage at which power consumption is minimized. The components selected to provide the correct voltage also affect the overall power consumption of the GNSS receiver: Low Dropout Regulator (LDO) - This solution is less expensive, but dissipates power as heat, so it is not conducive to optimizing power consumption. Switched Mode Power Supply (SMPS) is more efficient. However, this power supply uses a coil, which may cause unwanted RF interference in front of the antenna.
spare battery
A power outage causes the GNSS receiver to lose its position fix and all downloaded time and GNSS orbit data, so when power is restored the GNSS receiver is forced to do a complete cold start. By keeping this data in backup RAM, a GNSS receiver with a battery backup can recover from a power outage more quickly, saving power. When the position update cycle is longer than two hours (which roughly corresponds to the validity period of the ephemeris data), the backup battery becomes redundant and can be removed, reducing power consumption.
Real-time Clock (RTC)
Previous article:Blaize and LeiShen Intelligent Technology have reached a strategic cooperation agreement to integrate LiDAR and AI functions
Next article:TouchNetix Launches aXiom Touchless Sensor
Recommended ReadingLatest update time:2024-11-16 00:54
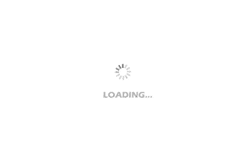
- Melexis launches ultra-low power automotive contactless micro-power switch chip
- Infineon's PASCO2V15 XENSIV PAS CO2 5V Sensor Now Available at Mouser for Accurate CO2 Level Measurement
- Milestone! SmartSens CMOS image sensor chip shipments exceed 100 million units in a single month!
- Taishi Micro released the ultra-high integration automotive touch chip TCAE10
- The first of its kind in the world: a high-spectral real-time imaging device with 100 channels and 1 million pixels independently developed by Chinese scientists
- Melexis Launches Breakthrough Arcminaxis™ Position Sensing Technology and Products for Robotic Joints
- ams and OSRAM held a roundtable forum at the China Development Center: Close to local customer needs, leading the new direction of the intelligent era
- Optimizing Vision System Power Consumption Using Wake-on-Motion
- Infineon Technologies Expands Leading REAL3™ Time-of-Flight Portfolio with New Automotive-Qualified Laser Driver IC
- LED chemical incompatibility test to see which chemicals LEDs can be used with
- Application of ARM9 hardware coprocessor on WinCE embedded motherboard
- What are the key points for selecting rotor flowmeter?
- LM317 high power charger circuit
- A brief analysis of Embest's application and development of embedded medical devices
- Single-phase RC protection circuit
- stm32 PVD programmable voltage monitor
- Introduction and measurement of edge trigger and level trigger of 51 single chip microcomputer
- Improved design of Linux system software shell protection technology
- What to do if the ABB robot protection device stops
- Allegro MicroSystems Introduces Advanced Magnetic and Inductive Position Sensing Solutions at Electronica 2024
- Car key in the left hand, liveness detection radar in the right hand, UWB is imperative for cars!
- After a decade of rapid development, domestic CIS has entered the market
- Aegis Dagger Battery + Thor EM-i Super Hybrid, Geely New Energy has thrown out two "king bombs"
- A brief discussion on functional safety - fault, error, and failure
- In the smart car 2.0 cycle, these core industry chains are facing major opportunities!
- The United States and Japan are developing new batteries. CATL faces challenges? How should China's new energy battery industry respond?
- Murata launches high-precision 6-axis inertial sensor for automobiles
- Ford patents pre-charge alarm to help save costs and respond to emergencies
- New real-time microcontroller system from Texas Instruments enables smarter processing in automotive and industrial applications
- What is wireless charging? What are the benefits of a wireless charging setup?
- PCB panelization skills
- A masterpiece by a senior Python programmer, helping you quickly master efficient Python programming
- MicroPython Hands-on (08) - Learn Color Recognition with MicroPython from Scratch
- Optical modules and 5G bearer networks
- Analysis of the switch input circuit composed of four diodes
- The power consumption evaluation data of a Bluetooth headset using Qualcomm CRS8635 is shared for discussion!
- Questions about TEC control chip ADN8834
- Amplifier input and output voltage range and rail-to-rail misunderstanding
- msp430f5529 simple uart source program (use the serial port assistant to send and receive replies)