This application note defines the thermocouple and explains its historical origins. The circuit described in this article digitizes the thermocouple output close to the temperature sensor, which minimizes noise compared to the solution of transmitting the weak signal through long cables before digitization. Thermocouples are widely
used in industry because of their high measurement accuracy, economic price, easy availability, and wide temperature measurement range. It consists of two different metal or metal alloy wires welded together (usually called the hot junction). The thermocouple output voltage is the difference between the two wire ends (the other end is usually called the cold junction), which must be maintained at a known temperature. Thermocouple voltage is a combination of the Seebeck (around 1921), Peltier (around 1834), and Thompson (around 1851) effects.
The terms hot junction and cold junction come from the history of applications. In fact, depending on the specific application, the cold junction temperature may be higher than the hot junction. In this case, the thermocouple output voltage is opposite. Therefore, the thermocouple measures the difference between the hot and cold junction temperatures, not the absolute temperature of the cold junction. Standard tables
have been prepared for the output voltages of thermocouples made of different metals or alloys.1 The standard metal pairs are represented by capital letters, for example, K for a nickel-cadmium thermocouple, and the data listed in the table assumes a cold junction temperature of 0°C. To obtain the absolute temperature of the hot junction, the cold junction temperature must be measured and the thermocouple output adjusted accordingly. This technique is called cold junction compensation. When thermocouples first came into use in the mid-1800s, absolute temperature measurements required the cold junction to be maintained at an equilibrium temperature of ice and distilled water to establish a true 0°C reference point. Thermocouple temperature sensors require special cables and connectors made of the same material as the thermocouple wire. As a result, commercial thermocouples are available in a variety of packages, sizes, and types, along with a complete selection of cables, connectors, and accessories.2,3 The cold junction isotherm is located at the input of the thermocouple signal processing module, which is usually mounted on a baseplate made of a high thermal conductivity material. The thermal conductivity of copper is 381 W/m°K (the same magnitude of change per degree in both Celsius and Kelvin). The input connections must be electrically isolated but thermally conductive to the baseplate. Ideally, the entire signal processing block should be kept in an isothermal environment. The signal processing circuitry consists of a low-voltage DC amplifier (thermocouple signal range is µV/°C), a temperature sensor, cold-junction compensation circuitry, an internal reference ADC, an open-thermocouple detector, an alarm indication, and a digital output interface. All of these functions are integrated into a small IC, such as the MAX6674 and MAX6675 , which only require external thermocouple and power connections. The serial output represents the temperature at the thermocouple sensing point. The MAX6674/MAX6675 internal thermocouple-to-digital converter circuit is ratiometric with nickel-cadmium alloy (type K) thermocouples. The MAX6674 measures 0 to +128°C with a resolution of 0.125°C; the MAX6675 measures 0 to 1024°C with a resolution of 0.25°C. Both ICs interface to a microcontroller or similar local intelligent circuit through an SPI™-compatible interface. If the sensing point is far from the controller, the thermocouple signal should be digitized near the sensing point. Like other low-voltage circuits, the thermocouple signal processing circuit is very sensitive to EMI. Thermocouple leads are often exposed to EMI (the level of interference noise picked up by the leads is proportional to the length of the leads). EMI increases the uncertainty of the received signal and reduces the accuracy of the temperature measurement. Special thermocouple cables are expensive to use for this environment, and it is difficult to determine the actual test temperature if other cables are used. To minimize noise, a control circuit can be used near the sensing point, and a remote control circuit can be added near the sensing point to provide local intelligence, introduce complex signal filtering and cable shielding. Figure 1 shows a better design to digitize the thermocouple output close to the sensing point. Figure 1. Power is provided at the far end of a 3000-foot cable, and the MAX6674/MAX6675 digitizes the thermocouple output close to the sensing point to minimize EMI. The SPI interface of the MAX6674/MAX6675 is driven by a local pulse timing generator (IC2 and IC3). IC2 and IC3 force the MAX6674/MAX6675 to generate asynchronous serial output data at a baud rate of 4800 and four characters per second. The character structure is: 1 start bit, 11 data bits, and 1 stop bit (MAX6675 uses 13 data bits). For the MAX6674, the 11-bit data includes 10 bits of direct binary numbers representing temperature data (MSB first) and 1 thermocouple open circuit alarm bit. The MAX6675 provides 12 bits of data and 1 alarm. A stable crystal oscillator ensures accurate data transmission baud rate. To ensure correct circuit operation, the thermocouple detection point must be electrically isolated from the circuit, and the MAX6674/MAX6675 must be kept within the operating temperature range of -20°C to +85°C at all times. The circuit is connected to the remote power supply and data receiver through twisted pair cables, and the circuit is powered and data is transmitted to the data receiver through the twisted pair cable. The temperature measurement is performed by the MAX6674/MAX6675's internal 10-bit ADC, which transmits the data serially over the cable. Figure 2 shows the temperature data generated by the MAX6674 and transmitted over 3000 feet of twisted-pair cable in quantized form. The data indicates that the thermocouple is in good working order, measuring a temperature of 21.875°C. Figure 2. The serial data word received at the data receiver A and B in Figure 1 represents the temperature measured by the thermocouple at the other end of the cable, which is 21.875°C.
Reference address:Quantify the thermocouple output near the sensor
used in industry because of their high measurement accuracy, economic price, easy availability, and wide temperature measurement range. It consists of two different metal or metal alloy wires welded together (usually called the hot junction). The thermocouple output voltage is the difference between the two wire ends (the other end is usually called the cold junction), which must be maintained at a known temperature. Thermocouple voltage is a combination of the Seebeck (around 1921), Peltier (around 1834), and Thompson (around 1851) effects.
The terms hot junction and cold junction come from the history of applications. In fact, depending on the specific application, the cold junction temperature may be higher than the hot junction. In this case, the thermocouple output voltage is opposite. Therefore, the thermocouple measures the difference between the hot and cold junction temperatures, not the absolute temperature of the cold junction. Standard tables
have been prepared for the output voltages of thermocouples made of different metals or alloys.1 The standard metal pairs are represented by capital letters, for example, K for a nickel-cadmium thermocouple, and the data listed in the table assumes a cold junction temperature of 0°C. To obtain the absolute temperature of the hot junction, the cold junction temperature must be measured and the thermocouple output adjusted accordingly. This technique is called cold junction compensation. When thermocouples first came into use in the mid-1800s, absolute temperature measurements required the cold junction to be maintained at an equilibrium temperature of ice and distilled water to establish a true 0°C reference point. Thermocouple temperature sensors require special cables and connectors made of the same material as the thermocouple wire. As a result, commercial thermocouples are available in a variety of packages, sizes, and types, along with a complete selection of cables, connectors, and accessories.2,3 The cold junction isotherm is located at the input of the thermocouple signal processing module, which is usually mounted on a baseplate made of a high thermal conductivity material. The thermal conductivity of copper is 381 W/m°K (the same magnitude of change per degree in both Celsius and Kelvin). The input connections must be electrically isolated but thermally conductive to the baseplate. Ideally, the entire signal processing block should be kept in an isothermal environment. The signal processing circuitry consists of a low-voltage DC amplifier (thermocouple signal range is µV/°C), a temperature sensor, cold-junction compensation circuitry, an internal reference ADC, an open-thermocouple detector, an alarm indication, and a digital output interface. All of these functions are integrated into a small IC, such as the MAX6674 and MAX6675 , which only require external thermocouple and power connections. The serial output represents the temperature at the thermocouple sensing point. The MAX6674/MAX6675 internal thermocouple-to-digital converter circuit is ratiometric with nickel-cadmium alloy (type K) thermocouples. The MAX6674 measures 0 to +128°C with a resolution of 0.125°C; the MAX6675 measures 0 to 1024°C with a resolution of 0.25°C. Both ICs interface to a microcontroller or similar local intelligent circuit through an SPI™-compatible interface. If the sensing point is far from the controller, the thermocouple signal should be digitized near the sensing point. Like other low-voltage circuits, the thermocouple signal processing circuit is very sensitive to EMI. Thermocouple leads are often exposed to EMI (the level of interference noise picked up by the leads is proportional to the length of the leads). EMI increases the uncertainty of the received signal and reduces the accuracy of the temperature measurement. Special thermocouple cables are expensive to use for this environment, and it is difficult to determine the actual test temperature if other cables are used. To minimize noise, a control circuit can be used near the sensing point, and a remote control circuit can be added near the sensing point to provide local intelligence, introduce complex signal filtering and cable shielding. Figure 1 shows a better design to digitize the thermocouple output close to the sensing point. Figure 1. Power is provided at the far end of a 3000-foot cable, and the MAX6674/MAX6675 digitizes the thermocouple output close to the sensing point to minimize EMI. The SPI interface of the MAX6674/MAX6675 is driven by a local pulse timing generator (IC2 and IC3). IC2 and IC3 force the MAX6674/MAX6675 to generate asynchronous serial output data at a baud rate of 4800 and four characters per second. The character structure is: 1 start bit, 11 data bits, and 1 stop bit (MAX6675 uses 13 data bits). For the MAX6674, the 11-bit data includes 10 bits of direct binary numbers representing temperature data (MSB first) and 1 thermocouple open circuit alarm bit. The MAX6675 provides 12 bits of data and 1 alarm. A stable crystal oscillator ensures accurate data transmission baud rate. To ensure correct circuit operation, the thermocouple detection point must be electrically isolated from the circuit, and the MAX6674/MAX6675 must be kept within the operating temperature range of -20°C to +85°C at all times. The circuit is connected to the remote power supply and data receiver through twisted pair cables, and the circuit is powered and data is transmitted to the data receiver through the twisted pair cable. The temperature measurement is performed by the MAX6674/MAX6675's internal 10-bit ADC, which transmits the data serially over the cable. Figure 2 shows the temperature data generated by the MAX6674 and transmitted over 3000 feet of twisted-pair cable in quantized form. The data indicates that the thermocouple is in good working order, measuring a temperature of 21.875°C. Figure 2. The serial data word received at the data receiver A and B in Figure 1 represents the temperature measured by the thermocouple at the other end of the cable, which is 21.875°C.
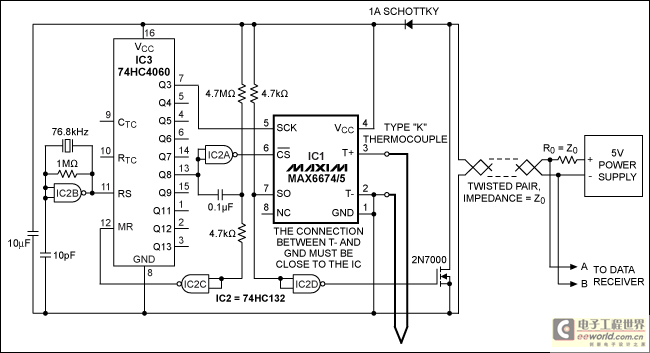
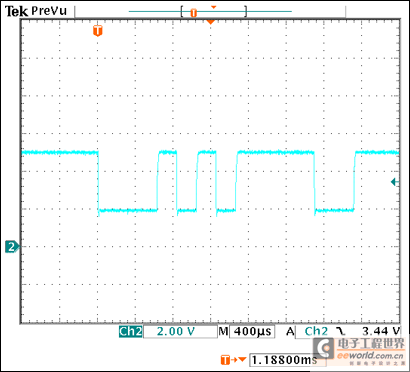
Previous article:Principle and design of water flow meter calibration control system
Next article:Design and application of image processing technology in part surface damage detection
- Popular Resources
- Popular amplifiers
Latest Industrial Control Articles
- Molex leverages SAP solutions to drive smart supply chain collaboration
- Pickering Launches New Future-Proof PXIe Single-Slot Controller for High-Performance Test and Measurement Applications
- CGD and Qorvo to jointly revolutionize motor control solutions
- Advanced gameplay, Harting takes your PCB board connection to a new level!
- Nidec Intelligent Motion is the first to launch an electric clutch ECU for two-wheeled vehicles
- Bosch and Tsinghua University renew cooperation agreement on artificial intelligence research to jointly promote the development of artificial intelligence in the industrial field
- GigaDevice unveils new MCU products, deeply unlocking industrial application scenarios with diversified products and solutions
- Advantech: Investing in Edge AI Innovation to Drive an Intelligent Future
- CGD and QORVO will revolutionize motor control solutions
MoreSelected Circuit Diagrams
MorePopular Articles
- Innolux's intelligent steer-by-wire solution makes cars smarter and safer
- 8051 MCU - Parity Check
- How to efficiently balance the sensitivity of tactile sensing interfaces
- What should I do if the servo motor shakes? What causes the servo motor to shake quickly?
- 【Brushless Motor】Analysis of three-phase BLDC motor and sharing of two popular development boards
- Midea Industrial Technology's subsidiaries Clou Electronics and Hekang New Energy jointly appeared at the Munich Battery Energy Storage Exhibition and Solar Energy Exhibition
- Guoxin Sichen | Application of ferroelectric memory PB85RS2MC in power battery management, with a capacity of 2M
- Analysis of common faults of frequency converter
- In a head-on competition with Qualcomm, what kind of cockpit products has Intel come up with?
- Dalian Rongke's all-vanadium liquid flow battery energy storage equipment industrialization project has entered the sprint stage before production
MoreDaily News
- Allegro MicroSystems Introduces Advanced Magnetic and Inductive Position Sensing Solutions at Electronica 2024
- Car key in the left hand, liveness detection radar in the right hand, UWB is imperative for cars!
- After a decade of rapid development, domestic CIS has entered the market
- Aegis Dagger Battery + Thor EM-i Super Hybrid, Geely New Energy has thrown out two "king bombs"
- A brief discussion on functional safety - fault, error, and failure
- In the smart car 2.0 cycle, these core industry chains are facing major opportunities!
- The United States and Japan are developing new batteries. CATL faces challenges? How should China's new energy battery industry respond?
- Murata launches high-precision 6-axis inertial sensor for automobiles
- Ford patents pre-charge alarm to help save costs and respond to emergencies
- New real-time microcontroller system from Texas Instruments enables smarter processing in automotive and industrial applications
Guess you like
- Ultra-low standby power consumption DC-DC controller chip
- Motor inductance problem
- I need a simulation diagram of a car reversing radar system (paid)
- Microcontroller touch dimming desk lamp program
- An interview question
- SVPWM Program
- Rumor has it that DJI is laying off 14,000 employees? PR Director: 14,000 in total
- About GDDFU Mode Application
- Summarize the commonly used communication interfaces of microcontrollers
- Considerations for using PMOS tube as power switch