Energy conservation and environmental protection are the main directions of current automotive technology research, and power batteries are the key to electric vehicle technology. Research on power battery characteristics is of great significance to electric vehicle batteries.
Lead-acid power batteries are low-cost, mature in technology, support large current discharge, and are highly safe. In non-power applications, a battery management system may not be required. However, their low specific energy cannot meet the range requirements of electric vehicles.
Nickel-hydrogen batteries and lithium-ion batteries are currently the main choices in the field of electric vehicle power batteries. Existing nickel-hydrogen batteries also have shortcomings, such as low single-cell voltage (1.2V), large self-discharge loss, and sensitivity to ambient temperature. The improvement of nickel-hydrogen batteries mainly focuses on improving performance and safety under high and low temperature conditions, optimizing charging and discharging performance, and reducing self-discharge. Compared with other batteries, lithium-ion batteries are used in electric vehicles and have greater advantages in energy and power performance. In terms of specific energy, discharge power, cycle life and sealing, they can meet the mid-term goals of the United States Advanced Battery Association (USABC) for electric vehicle power batteries.
1. Feature comparison
The types of power batteries studied in this article include lead-acid batteries, nickel-hydrogen batteries and lithium batteries.
1.1 Capacity characteristics
Capacity characteristics are the technical indicators that need to be examined first for power batteries. The main contents are the relationship between available capacity and discharge rate, and the change of available capacity at different temperatures. Due to the different rated capacities of batteries, the available capacity ratio is used in Figures 1 and 2:
Figure 1 Relationship between available capacity ratio and current
The available capacity is the amount of electricity discharged when the battery is discharged to the lowest cut-off voltage.
As shown in Figure 2, the available capacity of the 80 Ah nickel-hydrogen power battery is reduced because the battery temperature rises to 38°C when discharged under natural air cooling conditions. Similarly, for the VRLA65 Ah battery, the discharge process is terminated due to excessive temperature when discharged at a constant current of 400 A. On the contrary, the capacity characteristics of lithium-ion batteries are relatively ideal, the attenuation trend of the available capacity is not significant, and the available capacity does not change much; under the condition of lower rate current discharge, the lithium iron phosphate battery is higher than the lithium manganese oxide battery. Figure 2 shows the available capacity ratio of power batteries at different temperatures, and it can be seen that:
(1) The available capacity of lead-acid batteries, nickel-hydrogen batteries and lithium iron phosphate batteries is significantly affected by temperature;
(2) Batteries all have an optimal temperature range;
(3) The available capacity of lithium-ion batteries is relatively stable when affected by temperature, but the available capacity of lithium iron phosphate batteries is more sensitive to low temperatures.
Figure 2 Relationship between available capacity ratio and temperature
1.2 Efficiency characteristics
Efficiency is an important parameter of the battery and one of the key parameters in the process of estimating the remaining battery capacity. The measurement and calculation of coulombic efficiency shall be based on the USABC Battery Test Manual. Figures 3 and 4 show the coulombic efficiencies of VRLA batteries and lithium-ion batteries at different temperatures. It can be seen from Figures 3 and 4 that the coulombic efficiency of lead-acid batteries, nickel-hydrogen batteries and lithium-ion batteries is generally the same, with the following differences:
(1) The high temperature Coulombic efficiency of lead-acid batteries and lithium-ion batteries is higher than that of nickel-hydrogen batteries;
(2) The Coulombic efficiency of lithium-ion batteries is less correlated with temperature.
Figure 3 Coulombic efficiency of VRLA batteries at different temperatures
Figure 4 Coulombic efficiency of three lithium manganese oxide batteries at different temperatures
1.3 Voltage characteristics
The parameter matching of electric vehicle power system requires understanding of the voltage characteristics of power battery. This section uses modules with battery voltage between 10 and 12 V as the analysis object. The lead-acid battery module has 6 single cells connected in series, the nickel-hydrogen battery module has 10 single cells connected in series, and the lithium-ion battery module has 3 single cells connected in series.
(1) 10~12V module open circuit voltage
Figure 5 10-12V module-level battery open circuit voltage
From Figure 5, we can see that:
a. The VOC of lithium manganese oxide battery is higher than that of lithium iron phosphate battery, and its relationship with SOC is more similar to that of nickel hydrogen battery;
b The correlation between Voc and SOC of lithium iron phosphate battery is poor;
c There is a big difference in Voc between liquid manganese oxide lithium ion battery and polymer manganese oxide lithium ion battery, but the Voc variation law between the two liquid manganese oxide lithium ion batteries is basically the same.
(2) Discharge voltage of 10~12V module
FIG6 is a comparison of discharge voltage characteristics of different battery modules.
Figure 6 Comparison of discharge voltage of different power batteries at 10~12 V module level
From Figure 6, we can see that:
a. NiMH batteries have different operating voltage characteristics from lead-acid batteries and lithium-ion batteries. In the early and late stages of discharge, the voltage transition is "soft", which is conducive to SOC estimation based on voltage. The voltage platform time in the middle stage of discharge is shorter.
b The constant current discharge voltage curves of lithium manganese oxide battery and lead acid battery are relatively close, while the voltage platform feature of lithium iron phosphate battery is more obvious, but the platform voltage value is lower than that of lithium manganese oxide battery.
1.4 DC internal resistance characteristics
DC internal resistance is an important indicator for characterizing the performance and life status of power batteries. It determines the high-power discharge capability of the battery and affects the thermal characteristics and efficiency of the battery.
1.4.1 DC internal resistance under different impulse currents
Figure 7 Charge and discharge internal resistance of nickel-hydrogen 80Ah battery module
Figure 7 shows the internal resistance of the nickel-hydrogen battery module under different currents. As can be seen from Figure 7, for nickel-hydrogen batteries:
(1) Regardless of the charge and discharge rate, the effect of SOC on the internal resistance of the battery is in the shape of a saddle, with the bottom of the saddle near SOC = 60%;
(2) The area with smaller internal resistance of the battery is 50%~80%.
Figure 8 shows the variation of the DC internal resistance of a lithium iron phosphate battery cell during discharge, and the variation of the DC internal resistance during charge is similar to that of the lithium iron phosphate battery. For lithium iron phosphate batteries, both the charging internal resistance and the discharging internal resistance decrease as the SOC increases. As the current increases, the DC internal resistance at different SOCs gradually becomes consistent.
Figure 8 Relationship between the DC internal resistance of a lithium iron phosphate battery cell and different currents
1.4.2 DC internal resistance at different temperatures
Figure 9 shows the influence of temperature on the DC internal resistance of nickel hydrogen batteries. Figures 10 and 11 show the relationship between the DC internal resistance and temperature of lithium-ion batteries with different positive electrode materials (lithium manganese oxide and lithium iron phosphate). The following conclusions can be drawn from the figures:
Figure 9 Effect of temperature on internal resistance of nickel-hydrogen battery module
(1) Regardless of whether it is a nickel-hydrogen battery or a lithium battery, the DC internal resistance of the battery increases as the temperature decreases, and the influence rules are generally the same;
(2) Compared with nickel-hydrogen batteries, the internal resistance of lithium-ion batteries increases dramatically at low temperatures, which is the main reason for the decline in their low-temperature performance.
Figure 10 Relationship between DC internal resistance and temperature of 100 Ah lithium-ion battery of lithium manganese oxide
Figure 11 Relationship between DC internal resistance and temperature of 150 Ah lithium iron phosphate lithium-ion battery
1.5 Battery Thermal Characteristics
The electrochemical working principles of the three types of power batteries, lead-acid, nickel-hydrogen and lithium-ion, are completely different, and their thermal properties, tolerable high temperatures and optimal operating temperature ranges are also different.
The cut-off temperature of lithium iron phosphate battery is 75℃, lead acid battery is 60℃, lithium manganese oxide battery cut-off temperature is generally between 55 and 60℃, and the normal operating temperature range of nickel hydrogen battery is -30 to 55℃. Excessive temperature will accelerate battery aging, shorten the life span, and affect the basic characteristics and performance of the battery, such as available capacity, charging acceptance, charging and discharging efficiency, energy power performance, and safety issues.
This section only provides a preliminary discussion on the thermal characteristics of the battery based on the battery wall temperature.
During the discharge process of lithium-ion batteries, the heat generated by electrochemical reactions and ohmic heat work together to make the temperature rise faster during lithium-ion discharge. Figure 12 is a curve showing the temperature change of the battery wall during the discharge process of a power battery.
Figure 12 Temperature curve of power battery during discharge
It can be seen from Figure 12 that:
(1) The high-rate discharge performance of the VRLA 65 Ah lead-acid power battery is relatively good, and the temperature change curve is quite close to that of the 100 Ah lithium-ion battery at 400 A discharge;
(2) The discharge temperature change of nickel-hydrogen batteries is relatively gentle;
(3) When the manganese oxide lithium-ion batteries of the product system 100 Ah and 60 Ah are discharged at 300 A and 240 A respectively, the temperature change curves are the same, and the change of temperature relative to SOC is nearly consistent;
(4) The temperature rise rate of lithium iron phosphate batteries when discharged at a large current of 450 A and 3 C is relatively low.
1.6 Self-discharge characteristics
The measure of self-discharge characteristics is charge retention. Figure 13 shows the charge retention curves of two lithium-ion batteries and nickel-hydrogen batteries. The difference in initial SOC will affect the charge retention rate. The charge retention rate of lithium-ion batteries is very high. Among them, the charge retention rate of liquid lithium-ion 100 Ah battery (initial SOC 100%) is 96.4% after 146 days of open circuit storage, while the charge retention rate of nickel-hydrogen batteries has dropped to 80.42% after 28 days of open circuit storage. Therefore, the charge retention rate is an aspect that needs to be improved in nickel-hydrogen batteries.
Figure 13 Charge retention rate of nickel-hydrogen battery and lithium-ion battery
2 Performance Comparison
Energy and power performance is the main performance index that needs to be assessed in the use of power batteries for electric vehicles, and is closely related to the vehicle's driving range and its power: the specific energy of lead-acid batteries VRLA is the lowest, only about 35 Wh/kg, while hydrogen-nickel batteries and lithium-ion batteries increase in turn. Figure 14 shows a comprehensive examination of battery energy and power performance, including the specific energy of five power batteries at different power levels. Lead-acid batteries have strong high-current and high-rate discharge capabilities, but their specific energy and unit power levels are greatly reduced. The low specific energy of hydrogen-nickel batteries is due to their limited high-temperature tolerance. Under effective thermal management, the discharge power level of hydrogen-nickel batteries is not inferior, but their specific energy is still far behind that of lithium-ion batteries.
Figure 14 Relationship between mass specific energy and discharge power level
Lithium manganese oxide batteries are currently the power batteries commonly used in high-end electric vehicles.
As can be seen from Figure 14, lithium-ion batteries have the best discharge power capability and the best comprehensive energy-power performance.
3 Conclusion
Lead-acid batteries, nickel-hydrogen batteries and lithium batteries have their own characteristics in basic characteristics, and each has its own advantages when applied to electric vehicle power batteries, and each has its own suitable occasions. However, in terms of application prospects and performance improvement potential, lithium-ion batteries have the best comprehensive evaluation and are the direction of future development of electric vehicle power batteries.
Previous article:Fourteen tips to help your phone battery last longer
Next article:Introduction of common rechargeable batteries
Recommended ReadingLatest update time:2024-11-16 15:59
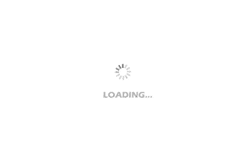
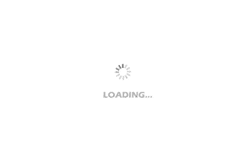
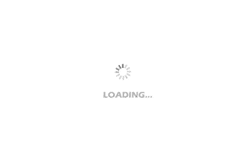
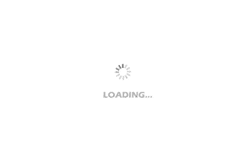
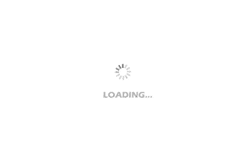
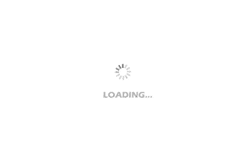
- MathWorks and NXP Collaborate to Launch Model-Based Design Toolbox for Battery Management Systems
- STMicroelectronics' advanced galvanically isolated gate driver STGAP3S provides flexible protection for IGBTs and SiC MOSFETs
- New diaphragm-free solid-state lithium battery technology is launched: the distance between the positive and negative electrodes is less than 0.000001 meters
- [“Source” Observe the Autumn Series] Application and testing of the next generation of semiconductor gallium oxide device photodetectors
- 采用自主设计封装,绝缘电阻显著提高!ROHM开发出更高电压xEV系统的SiC肖特基势垒二极管
- Will GaN replace SiC? PI's disruptive 1700V InnoMux2 is here to demonstrate
- From Isolation to the Third and a Half Generation: Understanding Naxinwei's Gate Driver IC in One Article
- The appeal of 48 V technology: importance, benefits and key factors in system-level applications
- Important breakthrough in recycling of used lithium-ion batteries
- Innolux's intelligent steer-by-wire solution makes cars smarter and safer
- 8051 MCU - Parity Check
- How to efficiently balance the sensitivity of tactile sensing interfaces
- What should I do if the servo motor shakes? What causes the servo motor to shake quickly?
- 【Brushless Motor】Analysis of three-phase BLDC motor and sharing of two popular development boards
- Midea Industrial Technology's subsidiaries Clou Electronics and Hekang New Energy jointly appeared at the Munich Battery Energy Storage Exhibition and Solar Energy Exhibition
- Guoxin Sichen | Application of ferroelectric memory PB85RS2MC in power battery management, with a capacity of 2M
- Analysis of common faults of frequency converter
- In a head-on competition with Qualcomm, what kind of cockpit products has Intel come up with?
- Dalian Rongke's all-vanadium liquid flow battery energy storage equipment industrialization project has entered the sprint stage before production
- Allegro MicroSystems Introduces Advanced Magnetic and Inductive Position Sensing Solutions at Electronica 2024
- Car key in the left hand, liveness detection radar in the right hand, UWB is imperative for cars!
- After a decade of rapid development, domestic CIS has entered the market
- Aegis Dagger Battery + Thor EM-i Super Hybrid, Geely New Energy has thrown out two "king bombs"
- A brief discussion on functional safety - fault, error, and failure
- In the smart car 2.0 cycle, these core industry chains are facing major opportunities!
- The United States and Japan are developing new batteries. CATL faces challenges? How should China's new energy battery industry respond?
- Murata launches high-precision 6-axis inertial sensor for automobiles
- Ford patents pre-charge alarm to help save costs and respond to emergencies
- New real-time microcontroller system from Texas Instruments enables smarter processing in automotive and industrial applications
- Free download of test solutions: R&SCMA180 - ideal test tool for analog and digital radio walkie-talkies
- Review summary: APM32E103VET6S MINI development board with the Polar Ocean M3 core
- Live broadcast at 13:15 this afternoon [Keysight World 2020|Telecom Infrastructure, Cloud and Artificial Intelligence Forum]
- [RISC-V MCU CH32V103 Review] - 4: EXTI starts the troubleshooting journey
- Use of SN74LVC125AD
- [Zero-knowledge ESP8266 tutorial] Quick Start 17 Station mode creates a wifi hotspot
- If I need to control about 3,000 lights in a room, is BLE MESH or zigbee more suitable?
- Example of connecting CANOpen gateway to Inovance inverter
- Which Bluetooth microphone has a reference circuit? Post it and discuss it
- How much does the Siemens 200smartPLC wireless communication module cost?