Direct heat pump
Direct heat pumps are designed to move heat energy from two radiators in two directions. This allows heat pumps to provide both heating and cooling functions in vehicles, which is actually achieved in two different architectures.
In the "air-to-air" (ATA) architecture, the refrigerant cycle works as a standard A/C system, and the HVAC module is modified to integrate an additional condenser, which replaces the traditional cabin heater.
In the air-to-water (ATW) architecture, the refrigerant cycle works as a standard A/C system with no modifications to the HVAC module, so a conventional cabin heater can be used thanks to the integration of a water-cooled condenser in the refrigerant circuit.
Both architectures are currently implemented in electric vehicles available on the market; in the following paragraphs, these systems are described in more detail.
Air-to-air (ATA) The layout of the heat pump system using the ATA structure is shown in Figure 3.
The air conditioning refrigerant circuit (green circuit in Figure 3) consists of the following components:
EVP: HEX (refrigerant/air heat exchanger) installed in the HVAC module
CHILLER: Required when refrigerant/coolant HEX is used to cool the battery coolant circuit;
ACOND: Refrigerant/Air HEX is installed in the HVAC module, it transfers heat to the cabin when needed;
OHX: Refrigerant/air external heat exchanger, designed for use as a condenser or evaporator;
EXV: Electronic Expansion Valve;
SOV: Stop valve with on/off control;
E-CMP: Electric compressor.
The coolant circuit (blue and purple in Figure 3) consists of the following components:
LT: Low temperature radiator, coolant/air HEX;
Figure 3 ATA heat pump architecture for pure electric vehicle applications. The standard HVAC module is modified to introduce an internal condenser (ACOND). A/C or heat pump function can be activated through dedicated stop valve (SOV) and expansion valve (EXV) control
Table 4 ATA valve arrangement under summer (A/C) and winter (heat pump) conditions
HV HTR: A high-voltage coolant heater dedicated to the battery heating function;
Dedicated coolant pump for battery and PWT circuits;
Coolant Valve: Three-way valve (V1 and V2) used to open or close the coolant path connecting the battery circuit to the LT.
In "hot summer conditions", the HP system works as a standard A/C system; the low temperature is transferred to the cabin via the EVP and to the battery via the cooler, the OHX works as a condenser, and the ACOND has no effect (but cannot be circumvented). In "cold winter conditions", the heat pump system achieves its true purpose: heat is transferred to the cabin via the ACOND and the OHX works as an evaporator to recover heat from the outside environment. Finally, in this case, if additional or faster heating heat is eventually needed, the HV PTC for the cabin and the HV coolant HTR for the battery are activated to achieve this.
Table 4 summarizes the EXV and SOV valve arrangements for the above two cases.
This architecture also allows waste heat to be recovered from the vehicle battery when it is necessary to heat the cabin and cool the battery simultaneously, such as during fast charging operations. In this case, the heat is lost from the battery via the cooler and is excluded from the cabin due to ACOND. To achieve this configuration, the EXV of the OHX is closed and SOV1 is open.
Air-to-Water (ATW) The layout of the heat pump system using ATW configuration is shown in Figure 4.
The air conditioning refrigerant circuit (green circuit in Figure 4) consists of the following components:
EVP: Refrigerant/Air HEX installed in HVAC module
CHILLER: Refrigerant/coolant HEX cools the battery coolant circuit when needed;
WCOND: Refrigerant/coolant HEX, transfers heat to the cabin when needed;
OHX: Refrigerant/air HEX, designed for use in condensers or evaporators;
EXV: Electronic Expansion Valve;
SOV: Stop valve with on/off control;
E-CMP: Electric compressor.
Figure 4 ATW heat pump architecture for pure electric vehicle applications. The standard HVAC module remains unchanged, but an additional plate heat exchanger (WCOND) is introduced. The air conditioning can be activated by controlling the dedicated stop valve (SOV) and the expansion valve EXV.
The coolant circuit (blue and purple in Figure 4) consists of the following components:
LT: Low temperature radiator, coolant/air HEX;
HV HTR: High-voltage coolant heater for battery and cabin heating;
Dedicated coolant pumps for cabin, battery and PWT circuits
Coolant Valves: Two three-way valves and one four-way valve to enable or disable the coolant path, connecting the cabin and battery return, or the PWT and battery return to the LT radiator.
In “summer or hot conditions”, the HP system works as a standard A/C system; the low temperature is transferred to the cabin through the EVP and to the battery through the cooler, the OHX works as a condenser, and WCOND has no effect (it is not bypassed by the refrigerant). In “winter cold conditions”, the heat pump system achieves its true purpose: heat is transferred to the coolant through WCOND through the refrigerant and released to the cabin through the CBN HTR. In this case, if additional or faster heating power is eventually required, the high-pressure PTC for the cabin and the high-pressure coolant HTR for the battery will be activated to achieve the target. Finally, in this case, the OHX works as an evaporator to recover heat from the external environment. The EXV and SOV valve arrangements for the above two cases are shown in Table 4, while the coolant valve (V1, V2 and V3) arrangements are shown in Tables 5 and 6.
As an ATA, the architecture can also recover waste heat from the vehicle battery when cabin heating and battery cooling are required simultaneously (e.g. during fast charging operations). In this case, the heat released from the battery, through the cooler, is discharged in the cabin thanks to WCOND and then CBN HTR. To achieve this configuration, the EXV of the OHX is closed, SOV1 is opened and the three-way valve on the cabin coolant circuit is closed towards the line connected to the battery.
In the ATA configuration, the ATW architecture can also recover waste heat from the electric motor through the coolant circuit. In this case, the active grille shutters at the front of the vehicle are closed, so the heat that would normally be rejected through the LT radiator can be used to heat the battery. To achieve this configuration, the three-way valve on the PWT coolant circuit is only open for the line connected to the battery, while the four-way valve is only open for the line connected to the PWT.
Table 5 ATW coolant valve arrangement
Table 6 ATW coolant valve arrangement under mixed working conditions
Virtual performance comparison
A mathematical model of the ATA and ATW system architectures was implemented using a commercial simulation software SW (AMESim17.0) in order to compare the performance of these HP solutions, taking into account the different functional objectives discussed in the previous paragraphs. In particular, a performance comparison was performed through an energy analysis that takes into account the energy consumption of all electrical components and the contribution of thermal energy exchanged with the different users, according to the following definition of system and HP COP:
The energy used by the user for cabin conditioning and battery conditioning is different:
Cabin Conditioning - The time integral of the change in enthalpy of air between the HVAC inlet and the cabin inlet.
Battery Conditioning – Changes in the Energy Stored Within a Battery
Through this analysis, the efficiency of the HP system is evaluated taking into account the boundary conditions described in Table 7 and taking a D-class electric vehicle as an example.
In the following paragraphs the results of the simulations are discussed ; the relative contributions of the different electrical components are discussed (Figures 13-16-18-20-23); these contributions can vary with changes in the system control strategy.
Table 7 Simulation boundary conditions
(1) Measure temperature @ cabin inlet (HVAC outlet)
(2) Coolant temperature @ chiller outlet, excluding battery heating target, where the reference is the battery temperature
Figure 5. Layout of the heat pump simulation model in AMESim. Each component of the HP is modeled as a block called a supercomponent. Each block is calibrated according to the detailed component data sheet.
Cabin cooling
In cabin cooling mode, the performance of the HP ATA architecture is slightly better than that of the ATW (ΔE ~ -3%, see Figure 6): at the same EVP outlet air temperature, the energy consumption is reduced and both the system and HP COP are increased due to the lower refrigerant pressure difference generated by the ACond heat exchanger relative to the Wcond heat exchanger.
Figure 6 Cabin cooling In terms of COP and energy consumption, the performance of the ATA architecture is slightly better than that of the ATW
Battery cooling (fast charging)
The worst case for battery cooling occurs during fast charging of the battery. A specific current profile was used to simulate this situation as shown in Figure 7 and implemented in the battery simulation model, considering the charge rate decreasing from 1.6 C at SOC = 0% of the battery to 0.72 C when the SOC increases to 80%.
During the simulation, until the heat released by the battery is higher than the cooling power of the chiller, the HP will not cool the battery but limit its temperature rise. Then, when the cooling potential of the chiller exceeds the heat release of the battery (up to a certain SOC value), the battery starts to cool (Figure 8).
During the simula ti on , until the battery thermal release is higher than the chiller cooling power, the HP does not cool down the battery, but limits its temperature increase. Then, when the chiller cooling potential overcomes the battery heat losses (up to a cert ai n SOC value), battery starts to cool down (Figure 8). The original text is here for fear of misunderstanding.
Previous article:A brief discussion on Bosch's cockpit monitoring technology
Next article:A brief discussion on the driving force of the development of the flat wire motor industry for new energy vehicles
Recommended ReadingLatest update time:2024-11-15 05:34
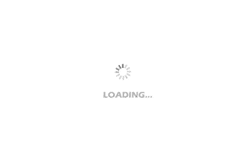
- Red Hat announces definitive agreement to acquire Neural Magic
- 5G network speed is faster than 4G, but the perception is poor! Wu Hequan: 6G standard formulation should focus on user needs
- SEMI report: Global silicon wafer shipments increased by 6% in the third quarter of 2024
- OpenAI calls for a "North American Artificial Intelligence Alliance" to compete with China
- OpenAI is rumored to be launching a new intelligent body that can automatically perform tasks for users
- Arm: Focusing on efficient computing platforms, we work together to build a sustainable future
- AMD to cut 4% of its workforce to gain a stronger position in artificial intelligence chips
- NEC receives new supercomputer orders: Intel CPU + AMD accelerator + Nvidia switch
- RW61X: Wi-Fi 6 tri-band device in a secure i.MX RT MCU
Professor at Beihang University, dedicated to promoting microcontrollers and embedded systems for over 20 years.
- LED chemical incompatibility test to see which chemicals LEDs can be used with
- Application of ARM9 hardware coprocessor on WinCE embedded motherboard
- What are the key points for selecting rotor flowmeter?
- LM317 high power charger circuit
- A brief analysis of Embest's application and development of embedded medical devices
- Single-phase RC protection circuit
- stm32 PVD programmable voltage monitor
- Introduction and measurement of edge trigger and level trigger of 51 single chip microcomputer
- Improved design of Linux system software shell protection technology
- What to do if the ABB robot protection device stops
- CGD and Qorvo to jointly revolutionize motor control solutions
- CGD and Qorvo to jointly revolutionize motor control solutions
- Keysight Technologies FieldFox handheld analyzer with VDI spread spectrum module to achieve millimeter wave analysis function
- Infineon's PASCO2V15 XENSIV PAS CO2 5V Sensor Now Available at Mouser for Accurate CO2 Level Measurement
- Advanced gameplay, Harting takes your PCB board connection to a new level!
- Advanced gameplay, Harting takes your PCB board connection to a new level!
- A new chapter in Great Wall Motors R&D: solid-state battery technology leads the future
- Naxin Micro provides full-scenario GaN driver IC solutions
- Interpreting Huawei’s new solid-state battery patent, will it challenge CATL in 2030?
- Are pure electric/plug-in hybrid vehicles going crazy? A Chinese company has launched the world's first -40℃ dischargeable hybrid battery that is not afraid of cold
- The basic working principle of electromagnetic slip clutch
- Are supercapacitors close to our lives?
- EEPROM address problem
- How many ohms are the resistors with silk screen 010 and 300?
- Compact lithium-ion secondary battery for IoT and wearable devices with both performance and safety
- The matching problem between op amp and ADC
- ZigBee-WSN temperature and humidity monitoring system
- Help: After selecting the package in AD09, no corresponding description appears
- TI HVI Power Technology Detailed 2019
- What is Ethernet? What is Industrial Ethernet?