Preface
The design of systems using high-speed analog-to-digital converters (ADCs) with high input frequencies (IFs) has always proven to be a challenging task. The use of transformers makes this task even more difficult because transformers have inherent nonlinearities that can make performance substandard. This article categorizes the issues that should be considered when designing high-speed sub-ranging ADCs using transformer-coupled front ends.
Design Parameters
There are several important parameters to consider when designing the front end.
The input impedance is the characteristic impedance of the design. In most cases it is 50Ω, but some designs require other impedance values. Transformers are essentially transimpedance devices because they can also couple circuits of different characteristic impedances when necessary so that the total system load is adequately balanced.
Bandwidth refers to the range of frequencies used by the system. This bandwidth can be narrow or wide, distributed over baseband (first Nyquist frequency), or covering multiple Nyquist zones.
Input drive level is a function of the bandwidth parameter and sets the system gain required for a particular application. Drive level is highly dependent on the front-end components used, such as filters and transformers, and this requirement can be one of the most difficult parameters to achieve.
The voltage standing wave ratio (VSWR) measures the amount of power reflected into the load over the bandwidth of interest. This parameter sets the input drive level required to achieve full-scale input to the ADC.
Passband flatness is the amount of fluctuation in performance within a specified bandwidth. This can be due to ripple effects or a very slow roll-off characteristic of a simple low-pass filter. Passband flatness is often less than or equal to 1dB and is critical to the overall system setup.
The signal-to-noise ratio (SNR) is the relationship between the signal seen by the converter and its own noise. In the front end, the SNR can be degraded due to bandwidth, signal quality (jitter), and gain. Remember, when the signal is amplified, the noise component is also amplified.
Spurious Free Dynamic Range (SFDR) is the ratio of the full-scale rms value to the rms value of the peak spurious spectral components. This is mainly due to two characteristics of the front end. The first characteristic is the linearity of the transformer, or the balance quality, which is related to the second harmonic distortion; the second characteristic is the matching relationship between the gain and the input. As the required gain increases, matching becomes more and more difficult, and the parasitic components of the nonlinear transformer (usually regarded as a third harmonic) will increase.
Transformer parameters
A transformer can be simply viewed as a bandpass filter.
Insertion loss, which represents the amount of loss in a transformer at a specific frequency, is the most common measurement parameter in a transformer data sheet, but it should not be the only consideration in a design.
Return loss is the transformer seen by the primary side when the secondary side of the transformer is terminated. For example, an ideal 1:2 impedance transformer with a 100Ω impedance on the secondary side will see a 50Ω impedance reflected on the primary side. However, this is not always true, as the impedance reflected by the primary side will change with frequency. As the impedance ratio increases, the return loss will also change accordingly.
Amplitude and phase imbalance is a key performance characteristic of transformers. When the design requires an IF frequency above 100MHz, these two specifications can give the designer an idea of how much nonlinearity may be encountered. As the frequency increases, the nonlinearity of the transformer also increases. Phase imbalance is often the main imbalance, which will result in even-order distortion or the increase of second harmonics.
ADC parameters
ADC can be divided into two types: buffered and unbuffered. The power consumption of unbuffered ADC is often much greater than that of buffered ADC, but buffered ADC is easier to drive.
The switched capacitor ADC is a specific example of an unbuffered ADC. The front-end design is directly connected to the sample-and-hold (SAH) network inside the ADC. This brings two problems: one is that the input impedance of the ADC changes over time and in different modes; the second is charge injection, which is reflected on the analog input of the ADC, which brings filter settling problems.
ADCs with buffers are the easiest to understand and use. Switching transients can be significantly reduced by using an isolation buffer that suppresses spikes from charge injection. Unlike the case in switched capacitor ADCs, the input termination characteristics do not change with analog input frequency over the entire specified ADC bandwidth, making the selection of an appropriate drive circuit more convenient. The downside of a buffered input stage is that it causes the ADC to consume more power.
Design Examples
Design examples for baseband and IF applications are given below and are shown in Figures 1 and 2, respectively.
In baseband applications, the input impedance of the ADC is generally high, so input matching is less important and easier to achieve. Often one or two small series resistors to attenuate charge injection effects plus simple differential input capacitors are sufficient. In this way, only a simple filter is needed to attenuate broadband noise for optimal performance.
Figure 1. Example of an unbuffered (switched capacitor) ADC
High frequency applications require a little more thought on the part of the designer. To optimize the input match, the input impedance needs to be made as resistive as possible by matching the front-end trace mode impedance. The "capacitive" term can be eliminated by using a series or parallel inductor or ferrite bead (the former is shown in the figure). The data sheets provided on each ADC product page on the www.analog.com website show the characteristics of an unbuffered ADC at various frequencies. Simply click on the Evaluation Board link on the product page to select an Excel spreadsheet that gives the characteristic impedance.
In summary, matching the input gives good bandwidth, gain flatness (less variation in power drive), and better performance (SFDR).
Figure 2: Example of a buffered ADC
Baseband applications with a buffered ADC also use a simple network similar to the switched capacitor ADC configuration. Note that the secondary side should be terminated to match the input of the primary side.
In Figure 2, a double balun (balanced/unbalanced conversion) is used for high IF applications. This allows the input to be well balanced up to 300MHz, keeping second-order distortion to a minimum.
summary
There are many parameters that must be considered in the design to achieve the best performance. Transformers vary widely. Designers who understand the specific transformer performance parameters and consult the manufacturer for parameters that are not given can better predict the characteristics of their design. High-IF designs are sensitive to transformer phase imbalance; therefore, these designs may require two transformers or a balun.
It is also important to know whether the ADC being used is buffered or unbuffered. The input impedance of an unbuffered ADC varies with time, making the design more difficult at high IFs. For optimal design, the inputs should be tracked and matched. Use ferrite beads or low-Q inductors to eliminate the input capacitance component of a switched capacitor ADC. This maximizes input bandwidth, allows for better input matching, and maintains SFDR performance. Buffered ADCs are easier to design with, even at high IFs, but they consume more power. Regardless of the ADC type used, baseband applications have the easiest design effort.
Previous article:Design of multi-channel AD sampling controller based on MPC5634
Next article:A Deeper Look at Difference Amplifiers
Recommended ReadingLatest update time:2024-11-16 16:56
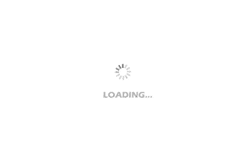
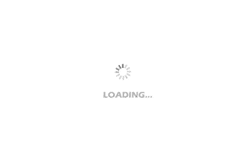
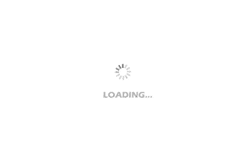
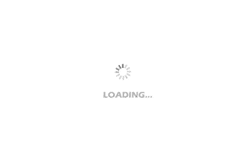
- Popular Resources
- Popular amplifiers
- MathWorks and NXP Collaborate to Launch Model-Based Design Toolbox for Battery Management Systems
- STMicroelectronics' advanced galvanically isolated gate driver STGAP3S provides flexible protection for IGBTs and SiC MOSFETs
- New diaphragm-free solid-state lithium battery technology is launched: the distance between the positive and negative electrodes is less than 0.000001 meters
- [“Source” Observe the Autumn Series] Application and testing of the next generation of semiconductor gallium oxide device photodetectors
- 采用自主设计封装,绝缘电阻显著提高!ROHM开发出更高电压xEV系统的SiC肖特基势垒二极管
- Will GaN replace SiC? PI's disruptive 1700V InnoMux2 is here to demonstrate
- From Isolation to the Third and a Half Generation: Understanding Naxinwei's Gate Driver IC in One Article
- The appeal of 48 V technology: importance, benefits and key factors in system-level applications
- Important breakthrough in recycling of used lithium-ion batteries
- Innolux's intelligent steer-by-wire solution makes cars smarter and safer
- 8051 MCU - Parity Check
- How to efficiently balance the sensitivity of tactile sensing interfaces
- What should I do if the servo motor shakes? What causes the servo motor to shake quickly?
- 【Brushless Motor】Analysis of three-phase BLDC motor and sharing of two popular development boards
- Midea Industrial Technology's subsidiaries Clou Electronics and Hekang New Energy jointly appeared at the Munich Battery Energy Storage Exhibition and Solar Energy Exhibition
- Guoxin Sichen | Application of ferroelectric memory PB85RS2MC in power battery management, with a capacity of 2M
- Analysis of common faults of frequency converter
- In a head-on competition with Qualcomm, what kind of cockpit products has Intel come up with?
- Dalian Rongke's all-vanadium liquid flow battery energy storage equipment industrialization project has entered the sprint stage before production
- Allegro MicroSystems Introduces Advanced Magnetic and Inductive Position Sensing Solutions at Electronica 2024
- Car key in the left hand, liveness detection radar in the right hand, UWB is imperative for cars!
- After a decade of rapid development, domestic CIS has entered the market
- Aegis Dagger Battery + Thor EM-i Super Hybrid, Geely New Energy has thrown out two "king bombs"
- A brief discussion on functional safety - fault, error, and failure
- In the smart car 2.0 cycle, these core industry chains are facing major opportunities!
- The United States and Japan are developing new batteries. CATL faces challenges? How should China's new energy battery industry respond?
- Murata launches high-precision 6-axis inertial sensor for automobiles
- Ford patents pre-charge alarm to help save costs and respond to emergencies
- New real-time microcontroller system from Texas Instruments enables smarter processing in automotive and industrial applications
- [Atria AT32WB415 Series Bluetooth BLE 5.0 MCU] + Bluetooth communication
- xilinx vivado xdc constraint syntax
- Design and Implementation of Digital Video Conversion Interface Based on FPGA
- [AT-START-F403A Evaluation] VI. FreeRTOS system based on IAR security library (sLib) secondary development mode practice
- About the 2021 E-Sports Championship
- [ST NUCLEO-G071RB Review]——First Look at the Kit
- FPGA Advanced Timing Synthesis Tutorial
- How reliable is Dupont wire???
- Classic Books on Signal Integrity Design
- How to avoid copper between the pins of AD18 chip