1 Overview
The DC-DC converter is the core component of the switching power supply, and the commonly used forward and flyback circuit topologies. The power processing circuit of the conventional forward converter has only one stage, and there is a large voltage stress on the MOSFET power switch, especially when the secondary side adopts the self-biased synchronous rectification method, and the input voltage variation range is wide. For example, when the input voltage is 75V, there is a risk that the gate bias voltage is too high, and there is even a risk that the synchronous rectification MOSFET may be damaged due to the gate voltage being too high. Moreover, when the output current is large, the loss on the output inductor will increase greatly, seriously affecting the improvement of efficiency. Using the cross-cascade forward synchronous rectification conversion circuit, not only the output filter inductor coil can be omitted, but also a high-efficiency and high-reliability DC-DC converter can be realized, achieving the best synchronous rectification effect.
2 Basic Technology
2.1 Principle of Cross-Cascade Forward Conversion
The topology of the cross-cascade conversion is shown in Figure 1. The synchronous buck converter consists of two-stage cross-cascade forward converters, with the front stage used for voltage stabilization and the back stage used for isolation. In order to achieve a wide input voltage range and constant voltage input to the isolation stage, both the front and rear forward converters should operate at optimal targets to ensure that the high-efficiency synchronous buck converter composed of them can accept the entire 35-75V communication input voltage range and convert it to a strictly regulated intermediate 25V bus voltage. The actual intermediate bus voltage is preset by the needs of the isolation stage and depends on the isolation stage ratio. When the intermediate voltage is higher, a smaller buck inductor value and lower inductor current can be used, resulting in less loss. The duty cycle of the entire buck stage is maintained at 30^'60%, which can help balance the losses of the front and rear forward converters. To optimize performance and minimize switching losses, the switching frequency is typically 240k-300kHz; due to the use of low on-state resistance (RDS(on)) MOSFETs, the conduction losses are relatively small. The traditional single-stage converter main switch must use a MOSFET of at least 200V, and its parameters such as RDS(on) are significantly increased, which inevitably means increased losses and reduced efficiency. The simplified schematic diagram of the cross-cascade forward converter topology is shown in Figure 2.
2.2 Synchronous Rectification Technology
As we all know, the forward voltage drop of an ordinary diode is 1V, and the forward voltage drop of a Schottky diode is 0.5V. When ordinary diodes and Schottky diodes are used as rectifiers, the power consumption of the rectifier itself is very considerable under high current conditions. In contrast, if a power MOSFET is used as a rectifier, when the driving voltage applied to the gate source of the MOSFET exceeds its threshold voltage, the MOSFET enters the on state, and can conduct current from the drain to the source or from the source to the drain. The voltage drop generated by the on-current on the MOSFET is only proportional to the channel resistance of the MOSFET. When n MOSFETs are connected in parallel, the voltage drop can be reduced to 1/n of a single MOSFET. Therefore, in theory, the loss caused by the voltage drop of the rectifier element can be artificially minimized. Synchronous Rectification (SR) is a technology that uses this characteristic of active devices such as MOSFET for rectification.
The main losses of implementing SR using power MOSFET are:
conduction loss: turn-on loss
: turn-off loss: drive loss: where I is the effective value of the forward current, RDS(on) is the on-state resistance, fS is the switching frequency, CGSS is the input capacitance, Coss is the output capacitance, and D is the duty cycle. It can be seen that the forward conduction loss is proportional to RDS(on). For MOSFETs with different VDS, RDS(on) can often differ by several orders of magnitude, so the loss of using 100V MOSFET in the same circuit topology is significantly lower than that of using 200V MOSFET. Considering that the Coss of low VDS MOSFET is smaller than that of high VDS MOSFET, according to the turn-off loss formula, it is shown that the turn-off loss of low VDS MOSFET is also small. The drive loss formula is the loss caused by the charging and discharging of the input capacitor during the switching process, which is proportional to the square of the gate-source drive voltage. Due to the use of a two-stage converter, for the isolation stage, the transformer ratio can be optimized because the voltage regulation stage has stabilized the wider input voltage at a fixed intermediate bus voltage. The forward on-state resistance RDS(on) and input capacitance of MOSFET are fixed, and the driving loss is only proportional to the square of the driving voltage. In short, the use of a two-stage converter can minimize the forward conduction loss, driving loss, etc. In addition, in the cross-cascade forward conversion circuit topology, the voltage required for the output stage synchronous rectification MOSFET is only twice the output voltage, plus a 1.2 times insurance factor, the device's withstand voltage is only 2.4 times the output voltage, which is much lower than the traditional single-stage converter solution that needs to reach 4-10 times the output voltage. In this way, the two-stage converter using the cross-cascade forward conversion circuit topology can use low-voltage, low RDS(on, MOSFET to achieve extremely low output stage conduction loss. The two-stage converter also uses the output of parallel MOSFETs to obtain lower RDS(on) and lower losses. When designing the overall system, as long as the heat distribution of the components is reasonable, the service life and reliability of the device will be greatly improved. 2.3 Current feedforward technology As can be seen from the figure, the secondary side of the cross-cascade forward converter circuit topology does not have an output filter inductor, while the single-stage converter must have an output filter inductor. When designing a single-stage converter, the discontinuous mode (DCM) and continuous mode (CCM) of the current in the output filter inductor must be taken into account. The selection of the inductance value is not only complicated in theoretical calculation, but also requires experimental verification. The isolation stage in the cross-cascade forward converter circuit topology adopts current feedforward technology, and the output filter inductor does not need to flow through the entire output current. Especially for low-voltage and high-current output, the output stage will not undergo unpredictable changes due to the increase in output current, which is the main advantage of this circuit topology. Therefore, when the system design needs to change proportionally, especially when the output voltage and output current change, the change in output current is already reflected in the input current of the primary isolation stage, which is the so-called current feedforward. In this way, the loss of the filter inductor is greatly reduced, thereby improving the efficiency of the converter. 3 Design examples and experimental results Applying the above design ideas, we designed a DC-DC half-brick power supply for communication equipment. The specific technical indicators are as follows: Input voltage DC3 5-75V: output voltage DC3.3V/30A; output power 100W; efficiency 92% (TYPICA); voltage regulation rate ±0.1%; load regulation rate ±0.1%; isolation voltage 1500V,,5; protection requirements are overvoltage, overcurrent, overtemperature, etc.
Figure 3 shows the schematic diagram of a DC-DC half-brick power supply for communication equipment designed with a cross-cascade forward conversion circuit. The working principle is as follows: R1, R2, D1, Q1, D2, and C1 form a bootstrap startup circuit to obtain a startup voltage Vc to power IC1, IC2, and IC3 respectively. After the circuit is started, the auxiliary winding of T1 is rectified by D3, and C3 smoothes and filters to provide a voltage VD for IC. Because the VD voltage is higher than Vc, the diode D2 is reverse biased, and the power supply of Q2 is turned off, achieving the purpose of zero power consumption of the startup circuit. Pin 6 of IC1 outputs a square wave signal, one of which is directly sent to pin 5 of ICl, and the other is sent to pin 6 of IC1 after being inverted by Q2 as the input signal of IC1. IC, pin 3 and pin 8 output square wave pulse signals with a phase difference of 180 degrees, respectively driving MOSFET Q31 Q4- Q3" Q4" L2 to form a high-efficiency synchronous buck stage, and the duty cycle of the buck stage is maintained at 30-60%. IC3.Qs"Q6"T. etc. form a cross-cascade forward isolation stage to achieve the final output voltage of DC-DC. Ma and DS are the magnetic reset windings of transformer T. Since the buck stage has strictly adjusted the input voltage with a wide range of variations to the intermediate bus voltage, the isolation stage does not need voltage regulation. The cross-cascade forward converters all operate at a duty cycle of 50%, and MOSFETs with a VDS of 100V can be used. Q7, Q: etc. form a self-biased synchronous rectification circuit. Because the output voltage of the isolation stage is fixed, the input voltage of the synchronous rectification MOSFET drain is also fixed, and the duty cycle is also 50%. A MOSFET with very low VDS can be used (in this example, a MOSFET with a VDS of 12V is used, which has the lowest loss). The heating problem caused by power consumption can be easily solved. Because the input voltage is fixed, when the output voltage is increased, high voltage regulation rate and high load regulation rate can be easily achieved, which is difficult for a single-stage converter to achieve. Other circuit functions (such as overcurrent, overvoltage, overtemperature protection, etc.) will not be explained one by one. After measurement, the working efficiency of the circuit is about 92%, which meets the predetermined design requirements, and the debugging is relatively simple, laying the foundation for future mass production.
4 Conclusion
The cross-cascade forward converter has a slightly complex circuit composition, but it can evenly distribute the losses of each stage to minimize the overall power consumption, so that it can work at a higher ambient temperature. Lower power consumption means higher efficiency; high operating ambient temperature means strong heat dissipation and large output current. The reduction in the cost of available output current indicates that the long-term reliability of the system will be better. Our practice shows that the cross-cascade forward synchronous rectification topology is indeed a very promising power conversion structure. All indicators are better than the same single-stage converter.
Reference address:Cross-cascade forward synchronous rectification topology to realize DC-DC converter
The DC-DC converter is the core component of the switching power supply, and the commonly used forward and flyback circuit topologies. The power processing circuit of the conventional forward converter has only one stage, and there is a large voltage stress on the MOSFET power switch, especially when the secondary side adopts the self-biased synchronous rectification method, and the input voltage variation range is wide. For example, when the input voltage is 75V, there is a risk that the gate bias voltage is too high, and there is even a risk that the synchronous rectification MOSFET may be damaged due to the gate voltage being too high. Moreover, when the output current is large, the loss on the output inductor will increase greatly, seriously affecting the improvement of efficiency. Using the cross-cascade forward synchronous rectification conversion circuit, not only the output filter inductor coil can be omitted, but also a high-efficiency and high-reliability DC-DC converter can be realized, achieving the best synchronous rectification effect.
2 Basic Technology
2.1 Principle of Cross-Cascade Forward Conversion
The topology of the cross-cascade conversion is shown in Figure 1. The synchronous buck converter consists of two-stage cross-cascade forward converters, with the front stage used for voltage stabilization and the back stage used for isolation. In order to achieve a wide input voltage range and constant voltage input to the isolation stage, both the front and rear forward converters should operate at optimal targets to ensure that the high-efficiency synchronous buck converter composed of them can accept the entire 35-75V communication input voltage range and convert it to a strictly regulated intermediate 25V bus voltage. The actual intermediate bus voltage is preset by the needs of the isolation stage and depends on the isolation stage ratio. When the intermediate voltage is higher, a smaller buck inductor value and lower inductor current can be used, resulting in less loss. The duty cycle of the entire buck stage is maintained at 30^'60%, which can help balance the losses of the front and rear forward converters. To optimize performance and minimize switching losses, the switching frequency is typically 240k-300kHz; due to the use of low on-state resistance (RDS(on)) MOSFETs, the conduction losses are relatively small. The traditional single-stage converter main switch must use a MOSFET of at least 200V, and its parameters such as RDS(on) are significantly increased, which inevitably means increased losses and reduced efficiency. The simplified schematic diagram of the cross-cascade forward converter topology is shown in Figure 2.

2.2 Synchronous Rectification Technology
As we all know, the forward voltage drop of an ordinary diode is 1V, and the forward voltage drop of a Schottky diode is 0.5V. When ordinary diodes and Schottky diodes are used as rectifiers, the power consumption of the rectifier itself is very considerable under high current conditions. In contrast, if a power MOSFET is used as a rectifier, when the driving voltage applied to the gate source of the MOSFET exceeds its threshold voltage, the MOSFET enters the on state, and can conduct current from the drain to the source or from the source to the drain. The voltage drop generated by the on-current on the MOSFET is only proportional to the channel resistance of the MOSFET. When n MOSFETs are connected in parallel, the voltage drop can be reduced to 1/n of a single MOSFET. Therefore, in theory, the loss caused by the voltage drop of the rectifier element can be artificially minimized. Synchronous Rectification (SR) is a technology that uses this characteristic of active devices such as MOSFET for rectification.
The main losses of implementing SR using power MOSFET are:
conduction loss: turn-on loss
: turn-off loss: drive loss: where I is the effective value of the forward current, RDS(on) is the on-state resistance, fS is the switching frequency, CGSS is the input capacitance, Coss is the output capacitance, and D is the duty cycle. It can be seen that the forward conduction loss is proportional to RDS(on). For MOSFETs with different VDS, RDS(on) can often differ by several orders of magnitude, so the loss of using 100V MOSFET in the same circuit topology is significantly lower than that of using 200V MOSFET. Considering that the Coss of low VDS MOSFET is smaller than that of high VDS MOSFET, according to the turn-off loss formula, it is shown that the turn-off loss of low VDS MOSFET is also small. The drive loss formula is the loss caused by the charging and discharging of the input capacitor during the switching process, which is proportional to the square of the gate-source drive voltage. Due to the use of a two-stage converter, for the isolation stage, the transformer ratio can be optimized because the voltage regulation stage has stabilized the wider input voltage at a fixed intermediate bus voltage. The forward on-state resistance RDS(on) and input capacitance of MOSFET are fixed, and the driving loss is only proportional to the square of the driving voltage. In short, the use of a two-stage converter can minimize the forward conduction loss, driving loss, etc. In addition, in the cross-cascade forward conversion circuit topology, the voltage required for the output stage synchronous rectification MOSFET is only twice the output voltage, plus a 1.2 times insurance factor, the device's withstand voltage is only 2.4 times the output voltage, which is much lower than the traditional single-stage converter solution that needs to reach 4-10 times the output voltage. In this way, the two-stage converter using the cross-cascade forward conversion circuit topology can use low-voltage, low RDS(on, MOSFET to achieve extremely low output stage conduction loss. The two-stage converter also uses the output of parallel MOSFETs to obtain lower RDS(on) and lower losses. When designing the overall system, as long as the heat distribution of the components is reasonable, the service life and reliability of the device will be greatly improved. 2.3 Current feedforward technology As can be seen from the figure, the secondary side of the cross-cascade forward converter circuit topology does not have an output filter inductor, while the single-stage converter must have an output filter inductor. When designing a single-stage converter, the discontinuous mode (DCM) and continuous mode (CCM) of the current in the output filter inductor must be taken into account. The selection of the inductance value is not only complicated in theoretical calculation, but also requires experimental verification. The isolation stage in the cross-cascade forward converter circuit topology adopts current feedforward technology, and the output filter inductor does not need to flow through the entire output current. Especially for low-voltage and high-current output, the output stage will not undergo unpredictable changes due to the increase in output current, which is the main advantage of this circuit topology. Therefore, when the system design needs to change proportionally, especially when the output voltage and output current change, the change in output current is already reflected in the input current of the primary isolation stage, which is the so-called current feedforward. In this way, the loss of the filter inductor is greatly reduced, thereby improving the efficiency of the converter. 3 Design examples and experimental results Applying the above design ideas, we designed a DC-DC half-brick power supply for communication equipment. The specific technical indicators are as follows: Input voltage DC3 5-75V: output voltage DC3.3V/30A; output power 100W; efficiency 92% (TYPICA); voltage regulation rate ±0.1%; load regulation rate ±0.1%; isolation voltage 1500V,,5; protection requirements are overvoltage, overcurrent, overtemperature, etc.

Figure 3 shows the schematic diagram of a DC-DC half-brick power supply for communication equipment designed with a cross-cascade forward conversion circuit. The working principle is as follows: R1, R2, D1, Q1, D2, and C1 form a bootstrap startup circuit to obtain a startup voltage Vc to power IC1, IC2, and IC3 respectively. After the circuit is started, the auxiliary winding of T1 is rectified by D3, and C3 smoothes and filters to provide a voltage VD for IC. Because the VD voltage is higher than Vc, the diode D2 is reverse biased, and the power supply of Q2 is turned off, achieving the purpose of zero power consumption of the startup circuit. Pin 6 of IC1 outputs a square wave signal, one of which is directly sent to pin 5 of ICl, and the other is sent to pin 6 of IC1 after being inverted by Q2 as the input signal of IC1. IC, pin 3 and pin 8 output square wave pulse signals with a phase difference of 180 degrees, respectively driving MOSFET Q31 Q4- Q3" Q4" L2 to form a high-efficiency synchronous buck stage, and the duty cycle of the buck stage is maintained at 30-60%. IC3.Qs"Q6"T. etc. form a cross-cascade forward isolation stage to achieve the final output voltage of DC-DC. Ma and DS are the magnetic reset windings of transformer T. Since the buck stage has strictly adjusted the input voltage with a wide range of variations to the intermediate bus voltage, the isolation stage does not need voltage regulation. The cross-cascade forward converters all operate at a duty cycle of 50%, and MOSFETs with a VDS of 100V can be used. Q7, Q: etc. form a self-biased synchronous rectification circuit. Because the output voltage of the isolation stage is fixed, the input voltage of the synchronous rectification MOSFET drain is also fixed, and the duty cycle is also 50%. A MOSFET with very low VDS can be used (in this example, a MOSFET with a VDS of 12V is used, which has the lowest loss). The heating problem caused by power consumption can be easily solved. Because the input voltage is fixed, when the output voltage is increased, high voltage regulation rate and high load regulation rate can be easily achieved, which is difficult for a single-stage converter to achieve. Other circuit functions (such as overcurrent, overvoltage, overtemperature protection, etc.) will not be explained one by one. After measurement, the working efficiency of the circuit is about 92%, which meets the predetermined design requirements, and the debugging is relatively simple, laying the foundation for future mass production.
4 Conclusion
The cross-cascade forward converter has a slightly complex circuit composition, but it can evenly distribute the losses of each stage to minimize the overall power consumption, so that it can work at a higher ambient temperature. Lower power consumption means higher efficiency; high operating ambient temperature means strong heat dissipation and large output current. The reduction in the cost of available output current indicates that the long-term reliability of the system will be better. Our practice shows that the cross-cascade forward synchronous rectification topology is indeed a very promising power conversion structure. All indicators are better than the same single-stage converter.
Previous article:A harmonic current detection method based on dq transformation
Next article:Termination of Clock Distribution Devices for High-Speed Converters
Recommended ReadingLatest update time:2024-11-16 23:44
Power Management: Meeting Complex DC-DC Power Conversion Requirements
introduction
In industrial applications such as test and measurement equipment or embedded computing, the system architecture of embedded DC-DC converters can be quite complex, with requirements on many different aspects such as output
voltage
and
current
, ripple, EMI, and power-up
[Power Management]
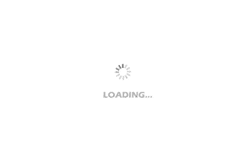
Research and design of DC-DC charge pump
1 Introduction The power supply is the heart of electronic equipment, and its quality directly affects the reliability of electronic equipment. With the continuous development of electronic technology, the requirements for power consumption, volume and conversion efficiency are constantly increasing, and switching c
[Power Management]
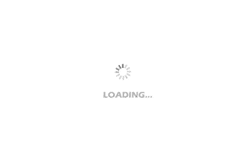
DC-DC Buck/Boost Regulator Design Solution
The job of a DC-DC switching converter is to efficiently convert one DC voltage to another. High-efficiency DC-DC converters use three basic technologies: buck, boost, and buck/boost. Buck converters are used to produce low DC output voltages, boost converters are used to produce high DC output voltages, and buck/bo
[Power Management]
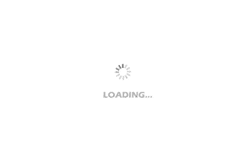
Design and implementation of PWM soft-switching DC-DC converter for on-board charger
For the on-board charging system, the design requirements of DC-DC converters are first pointed out, and the inherent deficiencies of traditional primary-side phase-shifted full-bridge DC-DC converters are analyzed. Then, the research progress of PWM soft-switching DC-DC converters in on-board chargers is detailed f
[Embedded]
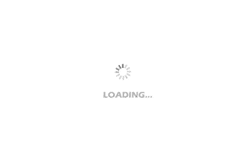
RAQ #214: Did you know that isolated DC-DC conversion does not require an optocoupler?
question:
How can optocoupler-free solutions help solve isolated DC-DC design challenges?
Answer:
Fortunately, there is a new optocoupler-free flyback DC-DC converter solution that eliminates the optocoupler and associated feedback circuitry and eliminates the n
[Power Management]
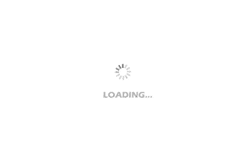
- Popular Resources
- Popular amplifiers
-
New Energy Vehicle Detection and Diagnosis Experiment (Edited by Mao Caiyun, Zhou Xien, and Long Jiwen)
-
Virtualization Technology Practice Guide - High-efficiency and low-cost solutions for small and medium-sized enterprises (Wang Chunhai)
-
Design of an adaptive constant on-time buck DC-DC converter with high efficiency and low power consumption
-
Research on design technology of high-efficiency step-up DC-DC converter with wide load range
Recommended Content
Latest Power Management Articles
- MathWorks and NXP Collaborate to Launch Model-Based Design Toolbox for Battery Management Systems
- STMicroelectronics' advanced galvanically isolated gate driver STGAP3S provides flexible protection for IGBTs and SiC MOSFETs
- New diaphragm-free solid-state lithium battery technology is launched: the distance between the positive and negative electrodes is less than 0.000001 meters
- [“Source” Observe the Autumn Series] Application and testing of the next generation of semiconductor gallium oxide device photodetectors
- 采用自主设计封装,绝缘电阻显著提高!ROHM开发出更高电压xEV系统的SiC肖特基势垒二极管
- Will GaN replace SiC? PI's disruptive 1700V InnoMux2 is here to demonstrate
- From Isolation to the Third and a Half Generation: Understanding Naxinwei's Gate Driver IC in One Article
- The appeal of 48 V technology: importance, benefits and key factors in system-level applications
- Important breakthrough in recycling of used lithium-ion batteries
MoreSelected Circuit Diagrams
MorePopular Articles
- Innolux's intelligent steer-by-wire solution makes cars smarter and safer
- 8051 MCU - Parity Check
- How to efficiently balance the sensitivity of tactile sensing interfaces
- What should I do if the servo motor shakes? What causes the servo motor to shake quickly?
- 【Brushless Motor】Analysis of three-phase BLDC motor and sharing of two popular development boards
- Midea Industrial Technology's subsidiaries Clou Electronics and Hekang New Energy jointly appeared at the Munich Battery Energy Storage Exhibition and Solar Energy Exhibition
- Guoxin Sichen | Application of ferroelectric memory PB85RS2MC in power battery management, with a capacity of 2M
- Analysis of common faults of frequency converter
- In a head-on competition with Qualcomm, what kind of cockpit products has Intel come up with?
- Dalian Rongke's all-vanadium liquid flow battery energy storage equipment industrialization project has entered the sprint stage before production
MoreDaily News
- Allegro MicroSystems Introduces Advanced Magnetic and Inductive Position Sensing Solutions at Electronica 2024
- Car key in the left hand, liveness detection radar in the right hand, UWB is imperative for cars!
- After a decade of rapid development, domestic CIS has entered the market
- Aegis Dagger Battery + Thor EM-i Super Hybrid, Geely New Energy has thrown out two "king bombs"
- A brief discussion on functional safety - fault, error, and failure
- In the smart car 2.0 cycle, these core industry chains are facing major opportunities!
- The United States and Japan are developing new batteries. CATL faces challenges? How should China's new energy battery industry respond?
- Murata launches high-precision 6-axis inertial sensor for automobiles
- Ford patents pre-charge alarm to help save costs and respond to emergencies
- New real-time microcontroller system from Texas Instruments enables smarter processing in automotive and industrial applications
Guess you like
- Do you know the power supply solutions for tethered, aerial/underwater drones? Vicor engineers tell you!
- Can anyone help me analyze this circuit?
- Electrician certification questions, increase daily knowledge
- How to learn FPGA technology?
- Announcement of Moderator Chip Coin and Physical Gift Rewards in November 2018
- Be the first to experience NUCLEO-WL55JC2, the world's first Lora SoC microcontroller!
- Brushless DC Motor
- 8 star products from industry leaders, listed on EDN Hot 100, let’s take a look
- Pimoroni Badger 2040 badge
- What inverter chip should I use for a 200Khz square wave signal?