Current sensing at the high side of a load is often desirable. However, engineers must overcome several design hurdles to successfully implement this approach. The circuit shown in Figure 1 has become a popular high-side dc sensing approach now that rail-to-rail op amps are available. This circuit is popular for several reasons. It operates from a single supply and has a wide supply voltage range, making it suitable for high-side or low-side current sensing. The input CMRR (common-mode rejection ratio) is also comparable to the basic rejection characteristics of the op amp itself and is not dependent on resistor matching. The gain and input and output voltage ranges of the circuit are easily set to achieve ±1% or better measurement accuracy. It also operates over a wide temperature range with proper component selection and does not require "special function" or single-supply ICs.
The advantage of the rail-to-rail op amps used in this example is that the input common-mode range goes all the way "to" the positive supply voltage. Most conventional op amps have an input voltage range of only about 1V or 2V of the positive supply voltage. Be careful when selecting a rail-to-rail op amp. Manufacturers may use this term to refer to the input voltage range, the output voltage range, or both. In this application, the key characteristic of a rail-to-rail op amp is that its input range includes the positive and negative voltages.
Source voltage. Some op amps are emerging that have this feature necessary for high-side sensing, although these devices are not classified as rail-to-rail op amps; an example is the LF355 FET-input op amp.
Figure 1 This basic DC current sensing circuit has good measurement accuracy, operates over a wide temperature range, and does not require a proprietary integrated circuit.
The circuit in Figure 1 senses the voltage drop across the current sense resistor and then adjusts the operating point of the output transistor to produce the same current in ROUT and in the feedback path formed by RIN. Since the transistor in this example has an inverting response, you must connect the feedback path back to the noninverting input to obtain a complete negative feedback response. The transfer function of this circuit is:
There are several potential sources of error in this circuit. Obviously, you want to make sure that the current sense resistor is as accurate as the application requires. Second, you want to make sure that the gain resistor (ROUT/RIN) is accurate. R'IN is optional to further reduce any offset errors caused by the input bias current of the op amp. In addition, you have to be careful with the output transistor. If you use a bipolar junction transistor, the additional base current will produce output errors. If the bipolar junction transistor has a beta of 100 (β=100), you can expect the output to increase by 1% (that is, 1/β, expressed as a percentage). You can greatly reduce this error by using Darlington transistors. Alternatively, you can use MOSFETs, which do not have this equivalent base current error because their source and drain currents are exactly the same. However, because of the potential for errors due to MOSFET leakage current, devices with low leakage current are the best choice. This leakage current only causes errors when measuring zero current or very small current values, but does not cause "gain" errors like bipolar junction transistors.
The last issue to address is input and output filtering. In most cases, adding a capacitor in parallel with ROUT is more than enough. Doing so will give the following familiar filtering response:
If the output must respond quickly to rapid changes in load current, you want to ensure that the output filter meets the necessary rise/fall time requirements. You can quickly estimate this value using the following formula:
If your circuit must operate in a harsh environment with high levels of radiated and conducted noise, make sure the sense pins are as short as possible and minimize the loop area between these pins. Do not connect a capacitor directly between the inverting and noninverting pins of the op amp; doing so may cause serious stability problems. If you insist on adding filtering at the input, use the arrangement shown in Figure 2. CDIFF helps limit the bandwidth of differential noise that would otherwise be amplified and processed as a legitimate signal. With the component values shown in Figure 2, the 3-dB bandwidth is approximately 800 Hz. Modifying the output stage (ROUT and COUT) can further improve filtering.
Figure 2 Input filtering allows a DC current sensing circuit to operate in a noisy environment.
Op amps can adequately reject common-mode noise, such as 60-Hz noise, but their ability to reject common-mode noise decreases with increasing frequency. In this regard, common-mode noise can be filtered out by adding common-mode capacitors (CCM) where the op amp is not effective. In the example given, f3dB-CM is set to approximately 160 kHz. Do not use a larger CCM to further improve common-mode rejection. If the CCM capacitor is too large and poorly matched, common-mode noise will be converted into a differential-mode signal (caused by the mismatch in capacitor value) and the amplifier will process it as a legitimate signal.
A rule of thumb is to choose the CDIFF value that makes the most sense for the set input bandwidth. Then, choose CCMs with capacitance as small as 1/10, preferably 1/100. And make sure these capacitors have reasonable tolerances and are well matched. Ceramic capacitors with very small temperature coefficients are inexpensive and very suitable for CCMs. Also, remember that proper decoupling between the op amp power pins is also very important because the conduction
Noise can also enter through the power supplies, especially when the op amp is powered directly from the high-side supply rail (as shown).
The output impedance of this circuit is equal to ROUT, so if you connect the output of this circuit to an ADC with relatively low input impedance (such as the ADC in many microcontrollers), you will need to use a follower to buffer the output to prevent unnecessary loading of the output and cause significant errors. Fortunately, if dual op amps are used, this task can be easily accomplished without increasing space by configuring the remaining op amp as a follower.
Full-swing op amps specified for ±15V supplies can be powered from supplies as high as 30V. The circuit shown in Figure 3 is ideal when powered from higher voltage supplies. The op amp only sees the voltage across the Zener regulator, while the output transistor provides the necessary ground-referenced level shifting. The only limitation on the maximum high-side voltage is that it must not exceed the rated breakdown voltage of the output transistor.
Figure 3. In the case of high-voltage DC current sensing, the Zener regulator provides a high-side reference supply for the op amp.
Note that the op amp's reference point is relative to its V+ supply terminal, whereas it was relative to its V- supply terminal in the previous circuit. This requires you to replace the pnp transistor (or p-channel FET) with an npn transistor (or n-channel FET) and observe the proper orientation of the inverting and non-inverting inputs. In other cases, the formulas, arguments, and other considerations that apply to the previous circuit apply.
, filtering techniques, and operating principles apply to this circuit. The only downside to this circuit is that it must use a separate op amp if the output requires buffering.
These circuits are flexible and generally have good performance. However, depending on the final choice of op amp, transistor, and filter circuit, it is always wise to test and ensure adequate compliance over the entire expected current measurement range. You can verify this stability by simply monitoring the base voltage or gate voltage with an oscilloscope as the circuit is subjected to a step change in load current.
Table 1 lists readily available current sensing integrated devices that have the same architecture as described in this article. However, while many of the devices listed in Table 1 may be "functionally equivalent" to each other, it is impossible to find any one that is not exclusively manufactured. When selecting such a device, make sure its voltage rating is within the design's supply voltage range. Some devices can perform bidirectional current sensing and may have a comparator to provide a "travel" indication. Some devices are actually rated for operation from -40°C to +125°C, expanding their range of applications in automotive and industrial applications.
The IR21XX series is unique in that its floating channel architecture allows high/low side current sensing at potentials up to 600V. Its output is not an analog voltage, but rather an output pulse width proportional to the current being measured. This allows microprocessor interfacing without an ADC. In addition, it can pass the pulse width through an optocoupler for true isolation.
Table 1 also lists some Hall effect type current sensing devices that can be used. Hall effect type current sensing can achieve complete isolation like a current transformer; the difference between the two is that the Hall effect is suitable for both DC and AC current sensing. The
devices listed in Table 1 are by no means complete, and many manufacturers regularly add current sensing devices to their product lines and retire some products at an even faster rate.
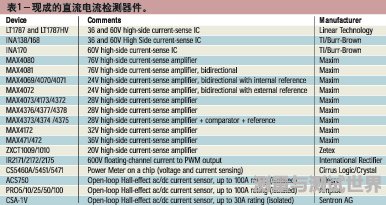
Previous article:Automotive Electronics ECU Development and Testing
Next article:The world of measurement and testing takes you into the automotive wind tunnel laboratory
- Popular Resources
- Popular amplifiers
- Seizing the Opportunities in the Chinese Application Market: NI's Challenges and Answers
- Tektronix Launches Breakthrough Power Measurement Tools to Accelerate Innovation as Global Electrification Accelerates
- Not all oscilloscopes are created equal: Why ADCs and low noise floor matter
- Enable TekHSI high-speed interface function to accelerate the remote transmission of waveform data
- How to measure the quality of soft start thyristor
- How to use a multimeter to judge whether a soft starter is good or bad
- What are the advantages and disadvantages of non-contact temperature sensors?
- In what situations are non-contact temperature sensors widely used?
- How non-contact temperature sensors measure internal temperature
- LED chemical incompatibility test to see which chemicals LEDs can be used with
- Application of ARM9 hardware coprocessor on WinCE embedded motherboard
- What are the key points for selecting rotor flowmeter?
- LM317 high power charger circuit
- A brief analysis of Embest's application and development of embedded medical devices
- Single-phase RC protection circuit
- stm32 PVD programmable voltage monitor
- Introduction and measurement of edge trigger and level trigger of 51 single chip microcomputer
- Improved design of Linux system software shell protection technology
- What to do if the ABB robot protection device stops
- Wi-Fi 8 specification is on the way: 2.4/5/6GHz triple-band operation
- Wi-Fi 8 specification is on the way: 2.4/5/6GHz triple-band operation
- Vietnam's chip packaging and testing business is growing, and supply-side fragmentation is splitting the market
- Vietnam's chip packaging and testing business is growing, and supply-side fragmentation is splitting the market
- Three steps to govern hybrid multicloud environments
- Three steps to govern hybrid multicloud environments
- Microchip Accelerates Real-Time Edge AI Deployment with NVIDIA Holoscan Platform
- Microchip Accelerates Real-Time Edge AI Deployment with NVIDIA Holoscan Platform
- Melexis launches ultra-low power automotive contactless micro-power switch chip
- Melexis launches ultra-low power automotive contactless micro-power switch chip
- Low-cost Wemos W600-PICO development board
- EEWORLD University Hall----American DIY players comprehensively test Keithley 2280S high measurement accuracy power supply
- Today at 10:00 AM, live broadcast with prizes: Melexis explains the implementation and technical support of semiconductor R&D functional safety
- Writing and running Python in the browser
- Technology Classroom | Cortex-M Architecture MCU Relocation Vector Table
- Book a live broadcast to get a gift: How to solve the coexistence problem in IoT medical devices
- CCS7.2 soft C28x chip software breakpoint setting method
- I learned C language from teacher Tan Haoqiang when I was in school, but I feel that the explanation is not comprehensive enough. Please recommend some tips for microcontroller programming.
- ADS8588S parallel byte mode read timing
- Design of intelligent remote control based on MSP430F413