Interfering with an electricity meter is as simple as placing a strong magnet near the power transformer. The applied magnetic field damages the power converter and hinders the meter's ability to accurately monitor power usage. The magnet can easily render the meter ineffective because the anti-interference schemes commonly implemented cannot detect it. There are no accurate figures to estimate how much power is stolen in this way, but industry experts believe that the problem is not to be underestimated and is worth preventing.
This article briefly reviews the physics behind magnetic interference in flyback power supplies and introduces a magnetic interference-resistant dual-output isolated flyback power supply design. The article concludes with a discussion of testing and performance comparisons between magnetic interference-resistant power supplies and conventional designs.
Ferrimagnetic and Magnet
The ferrimagnetic properties of transformer ferrite cores (ferrites have a crystal-random arrangement of magnetic pole pairs that can spontaneously rearrange) are a potential source of interference that often requires transformer redesign. Ferrite core materials are used in switch-mode transformers. When exposed to strong magnetic fields, the crystalline pole arrangement of the ferrimagnetic material changes. In power transformers, this can have knock-on effects on transformer (and power supply) performance:
1. The presence of an external magnetic field causes the internal field strength of the ferrite core to weaken
2. Flux density β decreases
3. The total magnetic flux is reduced, thereby reducing the primary inductance
4. If the external magnetic field is strong enough, the inductance will be reduced sufficiently, and the power supply will enter the auto-restart state as the switch current rises to its current limit before useful energy transfer occurs. In a circuit without a primary current limit, the possibility of switch failure is very high.
Power Integrations has developed a magnetically-protected, dual-output isolated flyback power supply that provides 16.5VDC@300mA and 16.5VDC@100mA output current over an input voltage range of 85 to 350VAC (Figure 1). Key design considerations include optimizing the transformer to compensate for the effects of external magnetic fields, as well as component selection and layout.
Figure 1: With a properly designed transformer, this flyback power supply can continue to operate normally in the presence of an external magnetic field.
The design is based on the LNK3696P of the LinkSwitch-XT2 900V product family . The LNK3696P IC integrates a 900V power MOSFET, an oscillator, a simple ON/OFF control method, a high-voltage switching current source, frequency modulation, cycle-by-cycle current limiting, and thermal shutdown, enabling a power solution with very few components.
Due to the ON/OFF control method, the output is checked before each switching cycle. If the output has dropped below the threshold limit, a switching cycle is initiated. If the output is within the limit, the switching cycle is skipped. During each switching cycle, the switch current is monitored as it rises, and the switching pulse is terminated when the current exceeds the preset limit. Therefore, each switching cycle is the same length and the total energy delivered to the load is fixed.
(figure 2).
Figure 2: Whenever the output voltage drops below the set limit, output power is delivered and measured by the feedback pin (VEN). In a short-circuit condition, the voltage will remain low and energy is required with every clock cycle.
If there are too many consecutive switching requests, a protective restart is triggered, reducing the output energy.
Because switching occurs only when needed, its light-load efficiency is higher than that of conventional PWM drivers.
The ON/OFF control method also does not require loop compensation because the design is a nonlinear system.
Power for startup and operation is derived directly from the DRAIN pin, eliminating the need for a bias winding and associated circuitry. At 100% load, when a harmful magnetic field is applied to the transformer of a conventional design, the LinkSwitch-XT2 is forced into an auto-restart state by the following process:
1. The applied magnetic field causes the primary inductance to drop by up to 50% of its rated value (1338μH)
2. As the inductance decreases, the core stores less and less energy in each switching cycle
3. To continue to support 100% power, the controller adds additional switching cycles to deliver less energy more frequently
4. A long series of continuous cycles at full frequency is interpreted as an output short circuit and a protective automatic restart is initiated. The power supply does not switch on and off normally - repeated attempts to restart are made.
To prevent auto-restart in the presence of an external magnetic field, the initial primary inductance needs to be doubled from 1338μH to 2676μH. Due to the ferrimagnetic effect mentioned above, applying an external magnetic field can reduce the inductance by up to 50% to 1338μH. With an inductance of 1338μH, the power supply can start and support 100% load without entering auto-restart.
Component Selection and Layout
Component selection and placement also help improve immunity to magnetic interference. To prevent any magnets from getting too close to the transformer, tall components (such as bulk capacitors, Y capacitors, and output capacitors) are placed around the transformer to form a barrier (Figure 3). Using a flat bobbin can also enhance the "component barrier" effect.
Test Methods
Figure 3: Example of a board showing DER-711 - Placing “tall” components around the transformer minimizes the risk of any external magnets approaching the transformer
The magnet used in the test can be moved toward the transformer from the side or top. In the test described here, a 6.35mm square N35 grade neodymium iron boron (NdFeB) magnet with a strength of 3451 gauss was placed next to the transformer core to create a "worst case" reduction of primary inductance by at least 50% (Figure 4). Under this test condition, the power supply was able to start up at 100% load and 75VAC input voltage (also the worst case).
Figure 4. Testing of the DER-711 PCB using a 3451 Gauss magnet placed directly next to the core (silver element in the red box).
Test Results and Performance Comparison
When designing a magnetically resistant power supply, you need to consider higher electromagnetic interference (EMI), longer startup time, lower power conversion efficiency, and possible audible noise. Placing the magnet on the core reduces the EMI margin required to meet the standard, and a magnetically resistant power supply design requires a larger EMI margin. DER-711 designs have a conducted EMI margin of >6dB.
As mentioned above, when an external magnetic field is placed near the core, the inductance is reduced, resulting in an increase in the switching frequency. The frequency of the relaxation oscillation of the drain voltage also increases accordingly. Shielded windings and proper input filter design are required to control the increased EMI caused by relaxation ringing. In addition to the EMI factor, the startup time is longer when the magnet is on the core, increasing from 9.68ms to 15.84ms (Figure 5).
Figure 5: In the presence of an external magnetic field, the startup time increases from 9.68ms to 15.84ms, an increase of approximately 6ms
In the extreme case where the magnet is directly adjacent to the transformer, the system efficiency has been tested to drop by only 4-6% (Figure 6). In these cases, the IC temperature increases by only 16°C at full load. If the applied magnetic field is weaker, the efficiency drop and the corresponding temperature rise are smaller.
Figure 6: In the worst case, placing the magnet next to the transformer reduces efficiency by only 4-6%.
The maximum flux density in this design is 2650 Gauss, which is significantly higher than the 1500 Gauss maximum recommended in the LinkSwitch-XT2 design. As a result, the switching frequency at full load is reduced and may be low enough to enter the audio noise range. This is not usually a disadvantage for power supplies in meter applications.
Summarize
An isolated flyback power supply that is immune to magnetic interference can be designed for an electricity meter with minimal design compromise. The key to the design is to increase the primary inductance to ensure that the power supply has enough margin to maintain uninterrupted operation at full load in the presence of external magnetic interference. At the same time, using a flat transformer skeleton and placing tall components around the transformer to form a "barrier" to minimize the proximity of external magnets to the transformer are also useful design strategies.
Previous article:TP8312 One or two dry cell battery boost IC solution to meet 0.9V low voltage operation
Next article:WPG Group launches 120W PD power solution based on ST products
Recommended ReadingLatest update time:2024-11-15 15:27
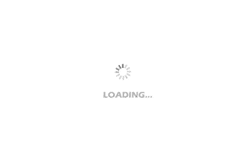
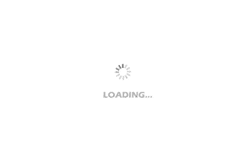
- Popular Resources
- Popular amplifiers
- MathWorks and NXP Collaborate to Launch Model-Based Design Toolbox for Battery Management Systems
- STMicroelectronics' advanced galvanically isolated gate driver STGAP3S provides flexible protection for IGBTs and SiC MOSFETs
- New diaphragm-free solid-state lithium battery technology is launched: the distance between the positive and negative electrodes is less than 0.000001 meters
- [“Source” Observe the Autumn Series] Application and testing of the next generation of semiconductor gallium oxide device photodetectors
- 采用自主设计封装,绝缘电阻显著提高!ROHM开发出更高电压xEV系统的SiC肖特基势垒二极管
- Will GaN replace SiC? PI's disruptive 1700V InnoMux2 is here to demonstrate
- From Isolation to the Third and a Half Generation: Understanding Naxinwei's Gate Driver IC in One Article
- The appeal of 48 V technology: importance, benefits and key factors in system-level applications
- Important breakthrough in recycling of used lithium-ion batteries
- LED chemical incompatibility test to see which chemicals LEDs can be used with
- Application of ARM9 hardware coprocessor on WinCE embedded motherboard
- What are the key points for selecting rotor flowmeter?
- LM317 high power charger circuit
- A brief analysis of Embest's application and development of embedded medical devices
- Single-phase RC protection circuit
- stm32 PVD programmable voltage monitor
- Introduction and measurement of edge trigger and level trigger of 51 single chip microcomputer
- Improved design of Linux system software shell protection technology
- What to do if the ABB robot protection device stops
- Learn ARM development(19)
- Learn ARM development(14)
- Learn ARM development(15)
- Analysis of the application of several common contact parts in high-voltage connectors of new energy vehicles
- Wiring harness durability test and contact voltage drop test method
- From probes to power supplies, Tektronix is leading the way in comprehensive innovation in power electronics testing
- From probes to power supplies, Tektronix is leading the way in comprehensive innovation in power electronics testing
- Sn-doped CuO nanostructure-based ethanol gas sensor for real-time drunk driving detection in vehicles
- Design considerations for automotive battery wiring harness
- Do you know all the various motors commonly used in automotive electronics?
- Excellent GaN PA recommendation, please take a look if you are interested.
- [GD32E503 Review]——step01. Start the journey of experiencing the domestically produced M33
- Practical sharing | How much do you know about the basics of laser technology?
- Apply for VICOR DCM DC-DC converter module related evaluation board for free!
- For thyristors, can digital resistors be used to control chopping in addition to optocouplers?
- ADI Award-winning Live Broadcast: Voltage Reference Product Technology and Application Selection July 25th 10:00-11:30 am Don't miss it~
- Why do you think that domestic chip manufacturers do not provide good support?
- 【ufun learning】Learning Part 3: "Basic Routine 2 - PWM Control"
- Last day! Prize-winning quiz to unlock the technology of Microchip's new MCUs!
- Today at 10:00 am TI Award Live Broadcast: Application of TI mmWave Sensors in Smart Home