The power supply is often the most easily overlooked part in the circuit design process. As an excellent design, the power supply design should be very important, which greatly affects the performance and cost of the entire system. The use of capacitors in power supply design is often the most easily overlooked part in power supply design.
1. Working principle of capacitor in power supply design
In power supply design applications, capacitors are mainly used for filtering and decoupling/bypassing. Filtering is the operation of filtering out specific frequency bands in a signal, and is an important measure to suppress and prevent interference. Based on the results of observing a random process, the probability theory and method of estimating another random process related to it. The term filtering originated from communication theory. It is a technique for extracting useful signals from received signals containing interference. The "received signal" is equivalent to the observed random process, and the "useful signal" is equivalent to the estimated random process.
Filtering mainly refers to filtering out external noise, while decoupling/bypassing (a kind of decoupling effect is achieved in the form of bypass, and "decoupling" will be used instead in the future) is to reduce the external noise interference of the local circuit. Many people tend to confuse the two. Let's look at a circuit structure below:
The power supply in the figure is for A and B. After the current passes through C1, it is divided into two paths through a section of PCB wiring to supply A and B respectively. When A needs a large current at a certain moment, if there is no C2 and C3, the voltage at the A end will become lower due to the line inductance, and the voltage at the B end will also be affected by the voltage at the A end and decrease. Therefore, the current change of the local circuit A causes the power supply voltage of the local circuit B, thereby affecting the signal of the B circuit. Similarly, the current change of B will also interfere with A. This is "common-path coupling interference."
After adding C2, when the local circuit needs a large current for a moment, capacitor C2 can temporarily provide current for A. Even if the common inductance exists, the voltage at the A end will not drop too much. The impact on B will also be greatly reduced. Therefore, the current bypass plays a role of decoupling.
Generally, large-capacity capacitors are mainly used for filtering. The speed requirement is not very fast, but the capacitance value requirement is relatively large. If the local circuit A in the figure refers to a chip, the capacitor should be as close to the power pin of the chip as possible. If "local circuit A" refers to a functional module, ceramic capacitors can be used. If the capacity is not enough, tantalum capacitors or aluminum electrolytic capacitors can also be used (the premise is that each chip in the functional module has a decoupling capacitor - ceramic capacitor).
The capacity of the filter capacitor can often be calculated from the data sheet of the power chip. If the filter circuit uses electrolytic capacitors, tantalum capacitors and ceramic capacitors at the same time, put the electrolytic capacitors as close to the power supply as possible to protect the tantalum capacitors. Put the ceramic capacitors behind the tantalum capacitors. This will achieve the best filtering effect.
Decoupling capacitors need to meet two requirements, one is the capacity requirement, the other is the ESR requirement. That is to say, the decoupling effect of a 0.1uF capacitor may not be as good as that of two 0.01uF capacitors. Moreover, 0.01uF capacitors have lower impedance in higher frequency bands. In these frequency bands, if a 0.01uF capacitor can meet the capacity requirement, it will have a better decoupling effect than a 0.1uF capacitor.
Many high-speed chip design manuals with many pins will give the requirements for decoupling capacitors in power supply design. For example, a BGA package with more than 500 pins requires a 3.3V power supply with at least 30 ceramic capacitors and several large capacitors, with a total capacity of more than 200uF...
Further reading: Capacitors and switching power supplies
2. Correct selection of capacitors in various power supplies
As a basic component, capacitors play an important role in electronic circuits. In traditional applications, capacitors are mainly used for bypass coupling, power supply filtering, DC isolation, oscillation in small signals, delay, etc. With the development of electronic circuits, especially power electronic circuits, different special requirements are put forward for capacitors in different applications.
Let’s start with the structure of a capacitor. The simplest capacitor is made up of plates at both ends and an insulating dielectric (including air) in the middle [1]. When electricity is applied, the plates become charged, forming a voltage (potential difference), but due to the insulating material in the middle, the entire capacitor is non-conductive. However, this is only possible if the critical voltage (breakdown voltage) of the capacitor is not exceeded. We know that any substance is relatively insulating. When the voltage across the two ends of a substance increases to a certain level, the substance can conduct electricity. We call this voltage the breakdown voltage.
Capacitors are no exception. After a capacitor is broken down, it is no longer an insulator. However, in middle school, such voltage is not seen in the circuit, so it works below the breakdown voltage and can be regarded as an insulator. However, in an AC circuit, the direction of the current changes with time in a certain functional relationship. The charging and discharging process of a capacitor takes time. At this time, a changing electric field is formed between the plates, and this electric field is also a function that changes with time.
1. Filter capacitor
After the alternating current (industrial frequency or high frequency) is rectified, it needs to be filtered by capacitors to make the output voltage smooth. The capacitor capacity is required to be large, and aluminum electrolytic capacitors are generally used. The main problem when using aluminum electrolytic capacitors is the relationship between temperature and life, which basically follows the 50℃ rule. Therefore, in many occasions requiring high temperature and high reliability, long-life (such as more than 5000h, even 105℃, 5000h) electrolytic capacitors should be selected. Generally, small-sized electrolytic capacitors have relatively short life.
Used in DC/DC switching power supply input filter capacitors. Since the switching converter draws power from the power supply in pulse form, a large high-frequency current flows through the filter capacitor. When the equivalent series resistance (ESR) of the electrolytic capacitor is large, a large loss will be generated, causing the electrolytic capacitor to heat up. Low ESR electrolytic capacitors can significantly reduce the heat generated by ripple (especially high-frequency ripple) current.
The electrolytic capacitor used for the output rectification of the switching power supply requires that its impedance frequency characteristics still do not show an upward trend at 300kHz or even 500kHz. However, ordinary electrolytic capacitors begin to show an upward trend after 100kHz, and the output rectification and filtering effect of the switching power supply is relatively poor. In the experiment, the author found that the ripple and peak of the ordinary CDII type 4700μF, 16V electrolytic capacitor used for the output filtering of the switching power supply are not lower than those of the CD03HF type 4700μF, 16V high-frequency electrolytic capacitor, and the temperature rise of ordinary electrolytic capacitors is relatively high. When the load is a sudden change, the transient response of ordinary electrolytic capacitors is far inferior to that of high-frequency electrolytic capacitors.
Since aluminum electrolytic capacitors cannot function well in the high frequency band, they should be supplemented with ceramic or non-inductive film capacitors with good high-frequency characteristics. Their main advantages are: good high-frequency characteristics and low ESR. For example, the MMK5 type 1μF capacitor has a resonant frequency of more than 2MHz and an equivalent impedance of less than 0.02Ω, which is much lower than that of electrolytic capacitors. Moreover, the smaller the capacity, the higher the resonant frequency (up to more than 50MHz), which will result in a good output frequency response or dynamic response of the power supply.
In the filter capacitor, we focus on how to choose the filter capacitor in the switching power supply.
How to choose filter capacitor for switching power supply
Filter capacitors play a very important role in switching power supplies. How to correctly select filter capacitors, especially output filter capacitors, is a matter of great concern to every engineering technician.
The pulsating voltage frequency of ordinary electrolytic capacitors used in 50 Hz power frequency circuits is only 100 Hz, and the charging and discharging time is in the order of milliseconds. In order to obtain a smaller pulsation coefficient, the required capacitance is as high as hundreds of thousands of microfarads. Therefore, the goal of ordinary low-frequency aluminum electrolytic capacitors is to increase the capacitance. The capacitance, loss tangent value and leakage current of the capacitor are the main parameters for distinguishing its quality. The output filter electrolytic capacitor in the switching power supply has a sawtooth voltage frequency of up to tens of thousands of hertz or even tens of megahertz. At this time, capacitance is not its main indicator. The standard for measuring the quality of high-frequency aluminum electrolytic capacitors is the "impedance-frequency" characteristic. It is required to have a lower equivalent impedance within the operating frequency of the switching power supply, and at the same time have a good filtering effect on the high-frequency spike signals generated when the semiconductor device is working.
Many electronic designers know the role of filter capacitors in power supplies. However, the filter capacitors used at the output end of switching power supplies are different from those used in industrial frequency circuits. The pulsating voltage frequency is only 100 Hz, and the charging and discharging time is in the order of milliseconds. In order to obtain a smaller ripple coefficient, the required capacitance is as high as hundreds of thousands of microfarads. Therefore, ordinary aluminum electrolytic capacitors are generally used to manufacture low-frequency capacitors. The main goal is to increase the capacitance. The capacitance, loss tangent and leakage current of the capacitor are the main parameters for distinguishing its quality.
The frequency of the sawtooth voltage on the electrolytic capacitor used as output filtering in the switching power supply is as high as tens of kilohertz or even tens of megahertz. Its requirements are different from those in low-frequency applications. Capacitance is not the main indicator. Its quality is measured by its impedance-frequency characteristics, which requires it to have low and constant impedance within the working frequency band of the switching power supply. At the same time, it can also have a good filtering effect on the peak noise of up to hundreds of kilohertz generated by the start of operation of semiconductor devices inside the power supply. Generally, ordinary electrolytic capacitors used for low frequencies begin to show inductive impedance at around 10 kilohertz, which cannot meet the requirements of switching power supplies.
Ordinary low-frequency electrolytic capacitors begin to show inductive properties at around 10,000 Hz, and cannot meet the requirements of switching power supplies. The high-frequency aluminum electrolytic capacitors used in switching power supplies have four terminals. The two ends of the positive aluminum sheet are respectively led out as the positive electrode of the capacitor, and the two ends of the negative aluminum sheet are also led out as the negative electrode. The current flows into one of the positive terminals of the four-terminal capacitor, passes through the capacitor, and then flows to the load from the other positive terminal; the current returning from the load also flows into one of the negative terminals of the capacitor, and then flows to the negative terminal of the power supply from the other negative terminal.
The high-frequency aluminum electrolytic capacitor used for switching power supplies has four terminals. The two ends of the positive aluminum sheet are led out as the positive electrode of the capacitor, and the two ends of the negative aluminum sheet are also led out as the negative electrode. The current of the voltage stabilized power supply flows into one positive terminal of the four-terminal capacitor, passes through the capacitor, and then flows to the load from the other positive terminal; the current returning from the load also flows into one negative terminal of the capacitor, and then flows to the negative terminal of the power supply from the other negative terminal. Because the four-terminal capacitor has good high-frequency characteristics, it provides an extremely advantageous means to reduce the pulsating component of the output voltage and suppress the switching spike noise.
The switching power supply has multifunctional comprehensive protection: In addition to the most basic voltage stabilization function, the voltage regulator should also have the most basic protection functions of overvoltage protection (exceeding +10% of the output voltage), undervoltage protection (lower than -10% of the output voltage), phase loss protection, and short-circuit overload protection. Spike pulse suppression (optional): The power grid sometimes has spike pulses with high amplitude and narrow pulse width, which will break down electronic components with low voltage resistance. The surge protection components of the stabilized power supply can effectively suppress such spike pulses.
High-frequency aluminum electrolytic capacitors also have multi-core forms, which divide the aluminum foil into several shorter segments and connect them in parallel with multiple lead sheets to reduce the resistance component in the capacitive reactance. At the same time, low-resistivity materials are used and screws are used as lead terminals to enhance the capacitor's ability to withstand large currents.
Stacked capacitors are also called non-inductive capacitors. Generally, the core of electrolytic capacitors is rolled into a cylindrical shape, and the equivalent series inductance is large. The structure of stacked capacitors is similar to that of books. The magnetic flux generated by the current flowing through is offset in the opposite direction, thereby reducing the inductance value and having better high-frequency characteristics. This type of capacitor is generally made into a square shape, which is easy to fix and can also appropriately reduce the volume occupied by the machine.
2. Absorption and commutation capacitors
As the rated power of gate-controlled semiconductor devices increases, the switching speed increases, and the rated voltage increases, it is not enough to simply require sufficient withstand voltage, capacity, and excellent high-frequency characteristics for the capacitors in the buffer circuit.
In high-power power electronic circuits, since the switching speed of IGBT is less than 1μs, it is normal to require the voltage change rate dv/dt on the absorption circuit capacitor to be > V/μs. Some circuits require V/μs or even V/μs.
For ordinary capacitors, especially ordinary metallized capacitors with dv/dt < 100V/μs, special metallized capacitors with dv/dt ≤ 200V/μs, special bimetallized capacitors with small capacity (less than 10nF) with dv/dt ≤ 1500V/μs, and large capacity (less than 0.1μF) with dv/dt ≤ 600V/μs, it is difficult to withstand such huge and high repetition rate peak current impact, which may damage power electronic circuits.
At present, the capacitors specially used in absorption circuits, i.e., metal foil electrodes, can withstand larger peak current and effective current impacts. For example, those with smaller capacity (below 10nF) can withstand a voltage change rate of 100,000V/μs to 455,000V/μs, a peak current of 3,700A and an effective current of 9A (such as CDV30FH822J03); those with larger capacity (greater than 10nF and less than 0.47μF) or larger size can withstand impacts of greater than 3,400V/μs and a peak current of 1,000A.
It can be seen that although they are all non-inductive capacitors, metallized capacitors and metal foil capacitors, they will have different performances when used in the absorption circuit. They are absolutely not interchangeable here because they have similar appearances but different specifications. The size of the capacitor will affect the dv/dt and peak current tolerance of the capacitor. Generally speaking, the larger the length, the smaller the dv/dt and peak current.
The working characteristics of the capacitor in the absorption circuit are high peak current with small duty cycle and low effective current. Similar to this circuit is the commutation capacitor of the thyristor inverter. Although the dv/dt required by this capacitor is smaller than that of the absorption capacitor, the peak current and effective current are both larger. Ordinary capacitors cannot meet the requirements in terms of current.
In some special applications, energy storage capacitors are required to discharge rapidly and repeatedly, and the discharge loop resistance is extremely low and the parasitic inductance is very small. In this case, only absorption capacitors can be used in parallel to ensure long-term reliability.
3. Resonant capacitor
Resonant converters, such as resonant switching power supplies and resonant capacitors in thyristor medium-frequency power supply resonant circuits, often flow large currents during operation. For example, if the specifications of the resonant capacitors of electronic ballasts are not properly selected, the capacitors may be damaged due to large resonant currents flowing through them even though the voltage on the capacitors does not reach the breakdown voltage.
In a circuit containing capacitors and inductors, if the capacitors and inductors are connected in parallel, it may happen that in a very short period of time: the voltage of the capacitor gradually increases, while the current gradually decreases; at the same time, the current of the inductor gradually increases, while the voltage of the inductor gradually decreases. In another very short period of time: the voltage of the capacitor gradually decreases, while the current gradually increases; at the same time, the current of the inductor gradually decreases, while the voltage of the inductor gradually increases. The increase in voltage can reach a positive maximum value, and the decrease in voltage can also reach a negative maximum value. Similarly, the direction of the current will also change in the positive and negative directions during this process. At this time, we call it electrical oscillation in the circuit.
The circuit oscillation phenomenon may gradually disappear, or it may continue to maintain unchanged. When the oscillation is maintained continuously, we call it constant amplitude oscillation, also known as resonance.
The time it takes for the voltage across a capacitor or inductor to change for one cycle is called the resonance period, and the inverse of the resonance period is called the resonance frequency. This is how the so-called resonance frequency is defined.
To sum up, in modern power supply technology, different applications require capacitors with different performances, which must not be mixed, abused, or misused, in order to eliminate unnecessary damage as much as possible and ensure product performance.
Further reading: Correct selection of capacitors in modern power supply technology
3. Capacitor step-down power supply design example
The conventional method of converting AC mains power into low-voltage DC is to use a transformer to step down the voltage and then rectify and filter it. When limited by factors such as size and cost, the simplest and most practical method is to use a capacitor step-down power supply.
1. Principle of capacitor step-down power supply circuit
The basic circuit of capacitor step-down simple power supply is shown in Figure 1. C1 is a step-down capacitor, D2 is a half-wave rectifier diode, D1 provides a discharge circuit for C1 during the negative half cycle of the mains, D3 is a voltage regulator diode, and R1 is the charge discharge resistor of C1 after the power is turned off. In practical applications, the circuit shown in Figure 2 is often used. When a large current needs to be provided to the load, the bridge rectifier circuit shown in Figure 3 can be used. The unregulated DC voltage after rectification is generally higher than 30 volts, and will fluctuate greatly with the change of load current. This is because the internal resistance of this type of power supply is large, so it is not suitable for applications with large current power supply.
2. Component selection principles for RC step-down circuits
(1) When designing a circuit, the exact value of the load current should be determined first, and then the capacity of the step-down capacitor should be selected based on the example. Excess current will flow through the Zener diode, and if the maximum allowable current Idmax of the Zener diode is less than Ic-Io, it may easily cause the Zener diode to burn out.
(2) To ensure reliable operation of C1, its withstand voltage should be greater than twice the power supply voltage.
(3) The selection of the discharge resistor R1 must ensure that the charge on C1 is discharged within the required time.
3. Design Examples
In Figure 2, it is known that C1 is 0.33μF and the AC input is 220V/50Hz. Find the maximum current that the circuit can supply to the load.
The capacitive reactance Xc of C1 in the circuit is: Xc=1/(2πfC)=1/(2*3.14*50*0.33*10-6)=9.65K
The charging current (Ic) flowing through capacitor C1 is: Ic = U / Xc = 220 / 9.65 = 22mA.
Usually, the relationship between the capacity C of the step-down capacitor C1 and the load current Io can be approximately considered as: C=14.5 I, where the capacity unit of C is μF and the unit of Io is A.
Previous article:Design of a New Intelligent Electronic Load
Next article:Solutions to the interference sources of the electromagnetic compatibility system of CAN bus communication
Recommended ReadingLatest update time:2024-11-16 22:47
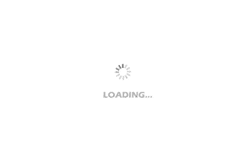
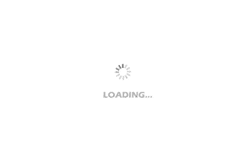
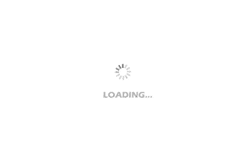
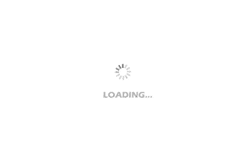
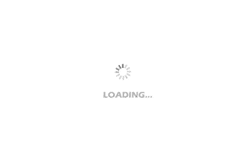
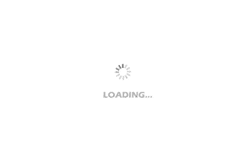
- MathWorks and NXP Collaborate to Launch Model-Based Design Toolbox for Battery Management Systems
- STMicroelectronics' advanced galvanically isolated gate driver STGAP3S provides flexible protection for IGBTs and SiC MOSFETs
- New diaphragm-free solid-state lithium battery technology is launched: the distance between the positive and negative electrodes is less than 0.000001 meters
- [“Source” Observe the Autumn Series] Application and testing of the next generation of semiconductor gallium oxide device photodetectors
- 采用自主设计封装,绝缘电阻显著提高!ROHM开发出更高电压xEV系统的SiC肖特基势垒二极管
- Will GaN replace SiC? PI's disruptive 1700V InnoMux2 is here to demonstrate
- From Isolation to the Third and a Half Generation: Understanding Naxinwei's Gate Driver IC in One Article
- The appeal of 48 V technology: importance, benefits and key factors in system-level applications
- Important breakthrough in recycling of used lithium-ion batteries
- Innolux's intelligent steer-by-wire solution makes cars smarter and safer
- 8051 MCU - Parity Check
- How to efficiently balance the sensitivity of tactile sensing interfaces
- What should I do if the servo motor shakes? What causes the servo motor to shake quickly?
- 【Brushless Motor】Analysis of three-phase BLDC motor and sharing of two popular development boards
- Midea Industrial Technology's subsidiaries Clou Electronics and Hekang New Energy jointly appeared at the Munich Battery Energy Storage Exhibition and Solar Energy Exhibition
- Guoxin Sichen | Application of ferroelectric memory PB85RS2MC in power battery management, with a capacity of 2M
- Analysis of common faults of frequency converter
- In a head-on competition with Qualcomm, what kind of cockpit products has Intel come up with?
- Dalian Rongke's all-vanadium liquid flow battery energy storage equipment industrialization project has entered the sprint stage before production
- Allegro MicroSystems Introduces Advanced Magnetic and Inductive Position Sensing Solutions at Electronica 2024
- Car key in the left hand, liveness detection radar in the right hand, UWB is imperative for cars!
- After a decade of rapid development, domestic CIS has entered the market
- Aegis Dagger Battery + Thor EM-i Super Hybrid, Geely New Energy has thrown out two "king bombs"
- A brief discussion on functional safety - fault, error, and failure
- In the smart car 2.0 cycle, these core industry chains are facing major opportunities!
- The United States and Japan are developing new batteries. CATL faces challenges? How should China's new energy battery industry respond?
- Murata launches high-precision 6-axis inertial sensor for automobiles
- Ford patents pre-charge alarm to help save costs and respond to emergencies
- New real-time microcontroller system from Texas Instruments enables smarter processing in automotive and industrial applications
- RS-485 communication interface encoder
- FPGA Implementation of Fully Parallel FFT
- RT-Thread has been ported to W600
- MakeCode now supports STM103
- Please help me with the trip zone configuration of TI's 28034!!!
- Is the threshold for electromagnetic wave and antenna major getting lower and lower? Is the reliance on simulation software getting higher and higher?
- EV-HC32F460_1. Unboxing
- The input capture of stm8s cannot enter the interrupt. Please help? ? Thank you!!!
- 10 bit serial controlled analog to digital converter (MS1549)
- [NXP Rapid IoT Review] I2C Program Small Bug