For designers selecting high-speed data converters, power consumption is the most important system design parameter. Whether it is a portable design that requires long battery life or a small product that consumes less heat, power consumption is critical. In the past, system designers have used low-noise linear regulators, such as low-dropout regulators, to power data converters instead of switching regulators because they were concerned that switching noise would enter the converter's output spectrum, greatly degrading AC performance.
However, a newer generation of noise-optimized switching regulators (used in cell phones) has enabled a shift in applications by minimizing interference with adjacent low-noise power amplifiers. They can power high-speed data converters directly from a DC/DC converter without significantly degrading AC performance. This design can immediately improve power efficiency by 20% to 25%.
Modern high-speed converters can consume about 50% less power than previous generations, in part by reducing the supply voltage from 3.3V to 1.8V. In a design using low-dropout regulators, as the supply rails go down, the regulator voltage drop and the available supply rails become more important to power efficiency. The data section of the board typically has many voltage rails, providing various core and I/O voltages for FPGAs and processors. In the analog section, there may be only a few "clean" voltages to choose from, such as 3.3V and 5V.
For a high-speed data converter, a linear regulator can be used to derive 3.3V from a common 5V rail. This results in a 1.7V drop across the low-dropout regulator, which translates to a power loss of about 35%. When a low-dropout regulator (such as the ADS4149) is used to provide 1.8V to an ADC from a 3.3V bus (Reference 1), the power loss in the linear regulator increases to about 45%, meaning that the low-dropout regulator dissipates almost half the power. This example shows how an inefficient power supply design can easily lose 50% of the power. The efficiency of a switching regulator is independent of the size of the input rail, so considerable power savings can be achieved. The impact on AC performance can be minimized through careful design.
Power supply filtering
A key component to isolate the switching noise from the ADC is the power supply filter, which includes a ferrite bead and bypass capacitors. Several key features should be considered when selecting a ferrite bead. First, the ferrite bead must have sufficient current rating for the data converter, and it must have a low DCR (DC resistance) to minimize the voltage drop across the bead itself. For example, when a 200mA power supply passes through a bead with a DCR of 1Ω, a 200mV voltage drop is generated. This voltage drop may push the voltage on the ADC to the edge, and considering the standard deviation of the power supply voltage, the ADC voltage may even be lower than the recommended operating voltage.
Secondly, the ferrite bead must have high impedance to the harmonics of the switching frequency and DC/DC converter to block switching noise and switching glitches. The impedance of most ferrite beads on the market is 100MHz, while the typical switching frequency of modern DC/DC converters is 500kHz~6MHz. In our example, the ADS4149 evaluation module uses a TPS625290 switching regulator with a switching frequency of 2.25MHz (Reference 2). Since the DC/DC regulator has a square wave output, higher-order harmonics must also be considered. Murata's NFM31PC276B0J3EMI filter has high impedance and low DCR in this frequency range.
Figure 1 compares the insertion loss of a traditional ferrite bead with a Murata EMI filter with a resistance of 68Ω at 100MHz. The power circuit has a low impedance, and the insertion loss is measured in a 50Ω environment. Therefore, the insertion loss value of the power filter may be slightly different even though the resonant frequency does not change.
Figure 1. Murata's NFM31PC276B0J3 EMI has high impedance and low DCR compared to a traditional ferrite bead with a resistance of 68Ω at 100 MHz.
The other components in the power filter are the bypass capacitors. These capacitor values should be chosen so that their resonant frequency (creating a low impedance path to ground) is close to the switching frequency. This way, the switching noise through the bead is shorted to ground. The power filter insertion loss comparison in Figure 2 shows that the correct bypass capacitor value can produce a resonance close to the switching frequency, even for a traditional ferrite bead, such as the EXCML32A680. However, at low frequencies, if it is placed together with a 0Ω resistor, there is not much difference. On the other hand, the Murata EMI filter provides about 20dB of additional attenuation around the switching frequency. The power filter in Figure 3 uses a 33μF tantalum capacitor for broadband decoupling, while the 10μF, 2.2μF, and 0.1μF ceramic capacitors have narrow resonant frequencies.
Figure 2. The correct bypass capacitor value can produce a resonance close to FS (switching frequency) even when used in conjunction with a traditional ferrite bead such as the EXC-ML32A680.
Figure 3: This power filter uses a 33μF tantalum capacitor for broadband decoupling, while 10μF, 2.2μF, and 0.1μF ceramic capacitors have narrow resonant frequencies.
AC Performance
Depending on the PSRR characteristics of the data converter, some amount of noise on the power rail can still enter the ADC and degrade the AC performance. Figure 4 shows a comparison of SNR and SFDR (spurious-free dynamic range) for a benchmark power supply (such as a 1.8V clean lab supply) using a low-dropout regulator and a DC/DC converter with different power supply filter options using the ADS4149 evaluation module.
Figure 4 These SNR (a) and SFDR (b) sweep plots compare a baseline power supply (such as a 1.8V clean lab supply) using a low dropout regulator and a DC/DC converter using the ADS4149 evaluation module with different power supply filter options.
The test results show that at an intermediate frequency of 300MHz, when powered by a switching regulator, the SNR performance is about 0.3dB lower than that of a low-noise LDO regulator. The SFDR performance is almost identical for all approaches. A careful look at the normalized FFT plot (starting with the input signal and plotting noise vs. offset frequency) shows that when using a suboptimal EXC ferrite bead, the background noise is slightly elevated throughout the Nyquist zone without any evidence of switching frequency feed-in (Figure 5).
Figure 5, a normalized FFT plot starting with the input signal and plotting noise vs. offset frequency, shows that when a suboptimal EXC ferrite is used, the background noise is slightly elevated across the Nyquist zone without any evidence of switching frequency feed-through.
Power efficiency
The main advantage of replacing linear regulators with DC/DC converters is energy savings. In all experiments with the ADS4149 evaluation module, an external 3.3V supply and a common analog power rail were used to power the LDO and switching regulators, respectively. Table 1 shows the measured power efficiencies, along with their corresponding quiescent currents. This comparison shows that the LDO almost always consumes much more power than the ADC. The switching regulator consumes only 32mW more power than an ideal solution, resulting in an efficient power supply design. When the input voltage is stepped down (from 3.3V to 2.5V or 2.2V), the efficiency of the LDO can be further improved, but at the expense of higher system cost and larger size.
Although a DC/DC converter design with a lower dropout voltage requires more external components, its overall footprint can be smaller because newer DC/DC converters have higher switching frequencies, which greatly reduces the size of the inductor, for example, 33μH is needed at 500kHz, while only about 2.2μH is needed at 2.25MHz.
Table 1: Comparison of converters
Conversely, linear regulators may not require power filtering, but they also have size limitations because they typically dissipate more power. From a cost perspective, switching regulators may cost slightly more because of the higher component count. However, the increased efficiency can offset the cost of cooling techniques and the system power budget (References 3 and 4).
As system designers seek more power efficient components, significant energy savings can be achieved by changing the power architecture in high-speed data converter designs to switching regulators. You can directly power a low-power, high-speed data converter with a switching regulator without significantly degrading its AC performance.
Previous article:Design Tips for Low-Power, Low-Cost Wireless ECG Monitors
Next article:Using the boost regulator above the rated voltage
Recommended ReadingLatest update time:2024-11-16 17:33
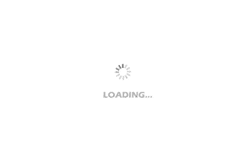
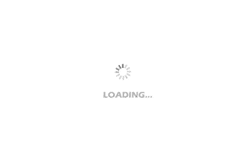
![[Lianshengde W806 Hands-on Notes] 10. ADC](https://6.eewimg.cn/news/statics/images/loading.gif)
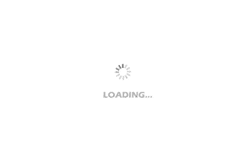
- Popular Resources
- Popular amplifiers
- MathWorks and NXP Collaborate to Launch Model-Based Design Toolbox for Battery Management Systems
- STMicroelectronics' advanced galvanically isolated gate driver STGAP3S provides flexible protection for IGBTs and SiC MOSFETs
- New diaphragm-free solid-state lithium battery technology is launched: the distance between the positive and negative electrodes is less than 0.000001 meters
- [“Source” Observe the Autumn Series] Application and testing of the next generation of semiconductor gallium oxide device photodetectors
- 采用自主设计封装,绝缘电阻显著提高!ROHM开发出更高电压xEV系统的SiC肖特基势垒二极管
- Will GaN replace SiC? PI's disruptive 1700V InnoMux2 is here to demonstrate
- From Isolation to the Third and a Half Generation: Understanding Naxinwei's Gate Driver IC in One Article
- The appeal of 48 V technology: importance, benefits and key factors in system-level applications
- Important breakthrough in recycling of used lithium-ion batteries
- Innolux's intelligent steer-by-wire solution makes cars smarter and safer
- 8051 MCU - Parity Check
- How to efficiently balance the sensitivity of tactile sensing interfaces
- What should I do if the servo motor shakes? What causes the servo motor to shake quickly?
- 【Brushless Motor】Analysis of three-phase BLDC motor and sharing of two popular development boards
- Midea Industrial Technology's subsidiaries Clou Electronics and Hekang New Energy jointly appeared at the Munich Battery Energy Storage Exhibition and Solar Energy Exhibition
- Guoxin Sichen | Application of ferroelectric memory PB85RS2MC in power battery management, with a capacity of 2M
- Analysis of common faults of frequency converter
- In a head-on competition with Qualcomm, what kind of cockpit products has Intel come up with?
- Dalian Rongke's all-vanadium liquid flow battery energy storage equipment industrialization project has entered the sprint stage before production
- Allegro MicroSystems Introduces Advanced Magnetic and Inductive Position Sensing Solutions at Electronica 2024
- Car key in the left hand, liveness detection radar in the right hand, UWB is imperative for cars!
- After a decade of rapid development, domestic CIS has entered the market
- Aegis Dagger Battery + Thor EM-i Super Hybrid, Geely New Energy has thrown out two "king bombs"
- A brief discussion on functional safety - fault, error, and failure
- In the smart car 2.0 cycle, these core industry chains are facing major opportunities!
- The United States and Japan are developing new batteries. CATL faces challenges? How should China's new energy battery industry respond?
- Murata launches high-precision 6-axis inertial sensor for automobiles
- Ford patents pre-charge alarm to help save costs and respond to emergencies
- New real-time microcontroller system from Texas Instruments enables smarter processing in automotive and industrial applications
- The best textbook for getting started with ADS (teaching you 9 experiments step by step)
- 【ST NUCLEO-G071RB Review】_06_DHT11 Temperature and Humidity Sensor Experiment
- BMW's new car uses electronic ink technology to change color with one click. Do you like it?
- Power supply temperature drift and ripple issues of constant current source
- Tesla launches a truck-shaped whistle for 318 yuan, mocking Apple's 145 yuan cleaning cloth
- How to enable TI 15.4-Stack to support 470M frequency band
- Design of temperature control system using DS18B20
- Introducing a touch capacitive screen IIC interface to USB solution
- ad16
- MOSFET switching voltage Vgs