First proposed in 1958, Class D amplifiers have become increasingly popular in recent years. So, what are Class D amplifiers? How do they compare to other types of amplifiers? Why do Class D amplifiers make sense for audio applications? What factors should be considered in designing a "good" Class D audio amplifier? What are the characteristics of Analog Devices' (ADI) Class D amplifier products? This article attempts to answer all of these questions.
Audio amplifier background
The purpose of an audio amplifier is to reproduce the input audio signal realistically, efficiently, and with low distortion at the sound-producing output element at the required volume and power level. The audio frequency range is approximately 20 Hz to 20 kHz, so the amplifier must have a good frequency response in this frequency range (reduced when driving a band-limited speaker, such as a woofer or tweeter). Output power capabilities vary widely depending on the application, from a few milliwatts (mW) for headphones, a few watts (W) for television (TV) or personal computer (PC) audio, tens of watts for "mini" home audio and car audio, to hundreds of watts and more for high-power home and commercial sound systems, as well as theater or concert hall sound systems.
A direct analog implementation of an audio amplifier uses transistors to produce an output voltage proportional to the input voltage in a linear mode of operation. The forward voltage gain is usually high (at least 40 dB). If the forward gain is part of a feedback loop, the overall loop gain will also be high. Feedback loops are often used because high loop gain improves performance, suppresses distortion due to linearity errors in the forward path, and reduces power supply noise by increasing power supply rejection (PSR).
Advantages of Class D Amplifiers
在传统晶体管放大器中,输出级包含提供瞬时连续输出电流的晶体管。实现音频系统放大器许多可能的类型包括A类放大器,AB类放大器和B类放大器。与D类放大器设计相比较,即使是最有效的线性输出级,它们的输出级功耗也很大。这种差别使得D类放大器在许多应用中具有显著的优势,因为低功耗产生热量较少,节省印制电路板( PCB )面积和成本,并且能够延长 便携式 系统的电池寿命。
Power consumption of linear amplifiers and Class D amplifiers
The linear amplifier output stage is connected directly to the speaker (in some cases through a capacitor). If bipolar junction transistors (BJTs) are used in the output stage, they are usually operated in a linear fashion with large collector-emitter voltages. The output stage can also be implemented with complementary metal oxide semiconductor ( CMOS ) transistors, as shown in Figure 1.
Figure 1. CMOS linear output stage.
Power is dissipated in all linear output stages because the process of generating the output voltage VOUT inevitably causes non-zero IDS and VDS in at least one of the output transistors. The amount of power dissipated depends primarily on the method used to bias the output transistors.
The Class A amplifier topology uses a transistor as a direct current (DC) current source to provide the maximum audio current required by the speaker. The Class A amplifier output stage can provide excellent sound quality, but the power consumption is very high because a large DC bias current usually flows through the output stage transistor (which is undesirable) and is not provided to the speaker (which is desirable).
The Class B amplifier topology has no DC bias current, so power consumption is greatly reduced. Its output transistors are independently controlled in a push-pull manner, allowing the high-side transistor to provide positive current to the speaker and the low-side transistor to absorb negative current. Since only the signal current flows through the transistor, the output stage power consumption is reduced. However, the sound quality of the Class B amplifier circuit is poor because linear errors (crossover distortion) are caused when the output current passes through zero and the transistor switches between the on and off states.
The Class AB amplifier is a combined compromise of the Class A and Class B amplifiers. It also uses a DC bias current, but it is much smaller than a pure Class A amplifier. The small DC bias current is sufficient to prevent crossover distortion, thereby providing good sound quality. Its power consumption is between that of the Class A and Class B amplifiers, but is usually closer to that of the Class B amplifier. Similar to the Class B amplifier circuit, the Class AB amplifier also requires some control circuitry to enable it to provide or sink large output currents.
Unfortunately, even a well-designed Class AB amplifier has significant power dissipation because its mid-range output voltage is usually far from the positive or negative supply. Due to the large voltage drop between the drain and source, a large instantaneous power dissipation IDS×VDS will be generated.
Class D amplifiers have a different topology (see Figure 2) and consume much less power than any of the above amplifiers. The output stage of a Class D amplifier switches between positive and negative supplies to generate a series of voltage pulses. This waveform helps reduce power consumption because the output transistor has zero current when it is not conducting and has a very low VDS when it is conducting, resulting in a smaller power consumption IDS×VDS.
Figure 2. Class D open-loop amplifier block diagram.
Since most audio signals are not pulse trains, a modulator must be included to convert the audio input into a pulse signal. The frequency content of the pulses includes the desired audio signal and important high-frequency energy associated with the modulation process. A low-pass filter is often inserted between the output stage and the speaker to minimize electromagnetic interference ( EMI ) and avoid driving the speaker with too much high-frequency energy. In order to maintain the power consumption advantages of the switching output stage, it is required that the filter (see Figure 3) be lossless (or nearly lossless). The low-pass filter usually uses capacitors and inductors , and the only energy-consuming element is the speaker.
Figure 3. Differential switching output stage and LC low-pass filter.
图4是A类放大器和B类放大器输出级功耗(PDISS)的理想值与AD1994 D类放大器输出级功耗的 测量 值的比较。图中的曲线是指给定的音频正弦波信号的输出级功率与扬声器提供的负载功率(PLOAD)之间的关系。其中负载功率相对最大负载(PLOAD max)功率水平归一化,箝位的正弦波信号保证10%总谐波失真(THD)。图中的垂直线表示PLOAD开始箝位的位置。
Figure 4. Comparison of power dissipation in the output stages of Class A, Class B, and Class D amplifiers.
可以看出,对于多种负载其功耗明显不同,尤其是在高端和中端值负载条件下。在箝位开始之初,D类放大器输出级的功耗约是B类放大器的1/2.5,是A类放大器的1/27。应当注意,消耗在A类放大器输出级的功率比传递到扬声器的功耗大,这是使用大的DC偏置电流的结果。
The output stage power efficiency Eff is defined as follows:
在箝位开始之初,A类放大器的Eff= 25%,B类放大器的Eff=78.5%,D类放大器的Eff=90%(见图5)。对于A类放大器和B类放大器,这些最佳例证经常在教科书中引用。
Figure 5. Comparison of power efficiency of Class A, Class B, and Class D amplifier output stages.
The difference in power consumption and power efficiency is large at moderate power levels. This is important for audio because the long-term average power level of loud music is much lower (1/5 to 1/20 of the instantaneous peak level that reaches PLOADmax , depending on the type of music). Therefore, for audio amplifiers, [PLOAD = 0.1 × PLOADmax] is a reasonable average power level at which to evaluate PDISS. At this power level, the power consumption of the output stage of a Class D amplifier is 1/9 of that of a Class B amplifier and 1/107 of that of a Class A amplifier.
For an audio amplifier with a 10 W PLOADmax, an average PLOAD of 1 W is considered a fidelity audio power level. Under this condition, the power dissipation within the output stage of a Class D amplifier is 282 mW, 2.53 W for a Class B amplifier, and 30.2 W for a Class A amplifier. In this case, the efficiency of the Class D amplifier is reduced from 90% at high power to 78%. But even 78% is much better than the Class B and Class A amplifiers, which have efficiencies of 28% and 3%, respectively.
These differences have important implications for system design. For power levels above 1 W, the excessive power dissipation of the linear output stage requires effective heat dissipation to avoid unacceptable heating, typically using a large metal plate as a heat sink or using a fan to promote air cooling through the amplifier. If the amplifier is an integrated circuit ( IC ), a large, high-cost thermally enhanced package may be required to promote heat conduction. These considerations are troublesome in consumer products such as flat-panel televisions , where printed circuit board area (PCB) area is at a premium, or in car audio, where the trend is to increase the number of channels in a fixed space.
For power levels below 1 W, dealing with wasted power can be more difficult than dealing with heat dissipation. If powered by a battery, a linear amplifier output stage consumes battery charge faster than a Class D amplifier. In the example above, the Class D amplifier output stage consumes 1/2.8 of the supply current of the Class B amplifier and 1/23.6 of the Class A amplifier, so they make a big difference in battery life in products such as cell phones, PDAs, and MP3 players.
So far, for simplicity, we have focused exclusively on the analysis of the amplifier output stage. However, when all power dissipation in the amplifier system is considered, linear amplifiers have an advantage over low-output-power Class D amplifiers. The reason is that at low power levels, the power required to generate and modulate the switching waveform can be significant. Therefore, the wide-system quiescent power dissipation advantage of well-designed low- and medium-power Class AB amplifiers makes them competitive with Class D amplifiers. Although for a wide range of output powers, there is no doubt that Class D amplifiers have the advantage of low power dissipation.
Class D amplifier terminology and comparison of differential and single-ended approaches
Figure 3 shows a differential implementation of the output transistors and LC filter in a Class D amplifier. This H-bridge has two half-bridge switching circuits that provide pulses of opposite polarity to the filter, which contains two inductors, two capacitors, and a speaker. Each half-bridge contains two output transistors, a high-side transistor MH connected to the positive supply and a low-side transistor ML connected to the negative supply. The high-side pMOS transistor is shown in Figure 3. High-side nMOS transistors are often used to reduce size and capacitance, but special gate drive methods are required to control them (see Further Reading 1).
The full H-bridge circuit is usually powered by a single power supply (VDD), and the ground terminal is used to connect the negative power supply terminal (VSS). For a given VDD and VSS, the differential method of the H-bridge circuit provides an output signal that is twice that of the single-ended method, and the output power is four times that of the single-ended method. The half-bridge circuit can be powered by a bipolar power supply or a unipolar power supply, but single-power supply will potentially damage the DC bias voltage because only VDD/2 voltage is applied to the speaker unless a DC blocking capacitor is added.
The supply voltage of the half-bridge circuit "excited" by the bus can exceed the nominal value produced by the large inductor current of the LC filter. Large decoupling capacitors between VDD and VSS can limit the transient changes of the excitation dV/dt. The full-bridge circuit is not affected by the bus excitation because the inductor current flows into one half-bridge and out of the other half-bridge, making the local current loop have minimal disturbance to the power supply.
Audio Class D Amplifier Design Factors
While there is a strong push to exploit the low power consumption of Class D amplifiers in audio applications, there are some important issues that designers need to consider, including:
● Output transistor size selection
●Output stage protection
Sound quality
●Preparation method
●Anti-electromagnetic interference (EMI)
● LC filter design
System cost
Output transistor size selection
The output transistor size is chosen to minimize power dissipation over a wide range of signal conditioning. Keeping VDS small while conducting a large IDS requires the output transistor's on-resistance (RON) to be small (typically 0.1Ω to 0.2Ω). This, however, requires a large transistor with a large gate capacitance (CG). The power dissipation in a switched capacitor gate drive circuit is CV2f, where C is the capacitance, V is the voltage change during charging, and f is the switching frequency. If the capacitance or frequency is too high, this "switching loss" will be too large, so there is a practical upper limit. Therefore, the choice of transistor size is a compromise between minimizing IDS × VDS losses during conduction and minimizing switching losses. At high output power, power dissipation and efficiency are dominated by conduction losses, while at low output power, power dissipation is dominated by switching losses. Power transistor manufacturers try to minimize the RON × CG of their devices to reduce the total power dissipation in switching applications, thereby providing flexibility in the choice of switching frequency.
Output stage protection
The output stage must be protected from a number of potentially hazardous conditions:
Overheating: Although Class D amplifier output stage power consumption is lower than that of linear amplifiers, if the amplifier provides very high power for a long time, it can still reach levels that harm the output transistors. To prevent dangerous overheating, temperature monitoring control circuits are needed. In a simple protection scheme, when the temperature measured by an on-chip sensor exceeds the thermal shutdown safety threshold, the output stage shuts down and remains shut down until it cools down. In addition to a simple binary indication of whether the temperature has exceeded the shutdown threshold, the sensor can provide other temperature information. By measuring the temperature, the control circuit can gradually reduce the volume level, reduce power consumption and keep the temperature well within the limit, rather than forcing silence during thermal shutdown.
Output transistor overcurrent: If the output stage and speaker terminals are properly connected, the output transistors will not be a problem in a low on-resistance state, but if these nodes are inadvertently shorted to another node or the positive or negative power supply, a huge current will be generated. If not checked, this current can damage the transistor or the surrounding circuit. Therefore, a current sensing output transistor protection circuit is required. In a simple protection scheme, if the output current exceeds a safe threshold, the output stage is shut down. In a more complex scheme.
The current sensor output is fed back into the amplifier, attempting to limit the output current to a maximum safe level while allowing the amplifier to continue operating without shutting down. In this scheme, forced shutdown is the last resort if current limiting protection is ineffective. An effective current limiter can also keep the amplifier operating safely during temporary large transient currents due to speaker resonance.
Undervoltage: Most switching output stage circuits can only operate properly when the positive supply voltage is high enough. If the supply voltage is too low, an undervoltage condition occurs, and problems arise. This problem is usually handled by an undervoltage lockout circuit that allows the output stage to operate only when the supply voltage is greater than the undervoltage lockout threshold.
Output Transistor Turn-On Timing: The MH and ML output stage transistors (see Figure 6) have very low on-resistance. Therefore, it is important to avoid the situation where both MH and ML are turned on at the same time, because it creates a low resistance path from VDD to VSS through the transistors, which can cause a large inrush current. In the best case, the transistors heat up and consume power; in the worst case, the transistors may be destroyed. Break-before-make control of the transistors prevents the inrush current situation from occurring by forcing both transistors to turn off before one transistor turns on. The time interval when both transistors are off is called the non-overlap time or dead time.
Figure 6. Make-before-break switching of output stage transistors.
Sound quality
In a Class D amplifier, several issues must be addressed to achieve good overall sound quality.
"Clicks and pops": Clicks and pops are very annoying when an amplifier is turned on or off. Unfortunately, they are easily introduced into Class D amplifiers unless careful attention is paid to the modulator state, output stage timing, and LC filter state when the amplifier is muted or unmuted.
Signal-to-Noise Ratio (SNR): To avoid hiss from the amplifier noise floor, the SNR should typically exceed 90 dB for low-power amplifiers for portable applications, 100 dB for medium- power designs , and 110 dB for high-power designs. This is achievable for a wide range of amplifiers, but specific noise sources must be tracked during amplifier design to ensure a satisfactory overall SNR.
Distortion Mechanisms: Distortion mechanisms include nonlinearities in the modulation technique or modulator implementation , and dead time used in the output stage to account for inrush current issues.
Information about the amplitude of the audio signal is usually encoded in the pulse width of the Class D modulator output. The additional dead time used to prevent current surges in the output stage introduces nonlinear timing errors that produce distortion at the speaker that is proportional to the timing error relative to the ideal pulse width. The shortest dead time to avoid surges is often the most beneficial for minimizing distortion; see Further Reading 2 for detailed design techniques to optimize distortion performance in switching output stages.
Other sources of distortion include mismatches in output pulse rise and fall times, mismatches in the timing characteristics of the output transistor gate drive circuits, and nonlinearities in LC low-pass filter components.
Power Supply Rejection (PSR): In the circuit shown in Figure 2, the power supply noise is coupled almost directly to the output speaker with little rejection. This occurs because the output stage transistors connect the power supply to the low-pass filter through a very low resistance. The filter rejects high frequency noise, but all audio frequencies are passed, including audio noise. See Further Reading 3 for a detailed description of the effects of power supply noise on single-ended and differential switching output stage circuits.
Without addressing distortion and power issues, it is difficult to achieve a PSR better than 10 dB or a total harmonic distortion (THD) better than 0.1%. Even worse, THD tends to high-order distortion that is detrimental to sound quality.
Fortunately, there are some good solutions to these problems. Using feedback with high loop gain (as is done in many linear amplifier designs) helps a lot. Feedback to the LC filter input greatly improves the PSR and attenuates all non-LC filter distortion sources. LC filter nonlinearities can be attenuated by including the speaker in the feedback loop. In a well-designed closed-loop Class D amplifier, high-fidelity sound quality with PSR > 60 dB and THD < 0.01% can be achieved.
But feedback complicates the design of the amplifier because loop stability must be satisfied (a complex consideration for high-order designs). Continuous-time analog feedback is also necessary to capture important information about pulse timing errors, so the control loop must include analog circuitry to process the feedback signal. In an integrated circuit amplifier implementation, this increases die cost.
To minimize IC cost, some manufacturers prefer to use no or minimal analog circuitry. Some use a digital open-loop modulator and an analog-to-digital converter to detect power supply variations and adjust the modulator behavior to compensate, see Further Reading 3. This improves PSR but does not address any distortion issues. Other digital modulators attempt to pre-compensate for expected output stage timing errors or correct for non-ideal modulators. This addresses at least some sources of distortion, but not all. For applications with relaxed sound quality requirements, these open-loop Class D amplifiers can handle this, but for the best sound quality, some form of feedback seems necessary.
Modulation Technology
Class D amplifier modulators can be implemented in many ways, with a large body of research and intellectual property supporting them. This article will only introduce the basic concepts.
All Class D amplifier modulation techniques encode information about the audio signal into a train of pulses. Typically, the pulse width is related to the amplitude of the audio signal, and the pulse spectrum includes the desired audio signal pulse and unwanted (but unavoidable) high-frequency content. The total integrated high-frequency power is roughly the same in all schemes, because the total power of the waveform is the same in the time domain, and according to Parseval's theorem, the power in the time domain must equal the power in the frequency domain. However, the energy distribution varies greatly: in some schemes, there are high-energy tones on top of a low noise floor, while in other schemes, the energy is shaped to eliminate the high-energy tones, but the noise floor is higher.
The most common modulation technique is pulse width modulation ( PWM ). In principle, PWM compares the input audio signal to a triangle or ramp wave operating at a fixed carrier frequency. This produces a train of pulses at the carrier frequency. The duty cycle of the PWM pulses is proportional to the amplitude of the audio signal during each carrier cycle. In the example of Figure 7, both the audio input and the triangle wave are centered around 0 V, so for zero input, the duty cycle of the output pulses is 50%. For large positive inputs, the duty cycle approaches 100%, and for large negative inputs, the duty cycle approaches 0%. If the audio amplitude exceeds the amplitude of the triangle wave, full modulation occurs, where the pulse train stops switching and the duty cycle is either 0% or 100% during a specific cycle.
PWM is attractive because it allows 100 dB or better audio-band SNR at PWM carrier frequencies of a few hundred kilohertz (low enough to limit output-stage switching losses). Many PWM modulators are also stable up to nearly 100% modulation, allowing, in principle, high output powers, up to the point of overload. However, PWM has several problems: first, the PWM process adds inherent distortion in many implementations (see Further Reading 4); second, resonances of the PWM carrier frequency can generate EMI in the amplitude-modulated (AM) radio band; and finally, the PWM pulse width is very small near full modulation. This causes problems in most switching output-stage gate-drive circuits, because their drive capabilities are limited and they cannot switch properly at the very fast speeds required to reproduce the short pulse widths of a few nanoseconds (ns). As a result, full modulation is often not achieved in PWM-based amplifiers, and the maximum achievable output power is less than the theoretical maximum, considering only supply voltage, transistor on-resistance, and speaker impedance.
An alternative to PWM is pulse density modulation (PDM), where the number of pulses in a given time window (pulse width) is proportional to the average value of the input audio signal. The individual pulse widths are not arbitrary as in PWM, but are "quantized" multiples of the modulator clock period. 1-bit sigma-delta modulation is a form of PDM.
A large amount of high-frequency energy in Σ-Δ modulation is distributed over a wide frequency range, rather than concentrated at multiples of the carrier frequency like PWM, so the potential EMI advantage of Σ-Δ modulation is better than PWM. Energy still exists at the mirror frequency of the PDM sampling clock frequency; but in the typical clock frequency range of 3 MHz to 6 MHz, the mirror frequency falls outside the audio frequency band and is strongly attenuated by the LC low-pass filter.
Another advantage of Σ-Δ modulation is that the minimum pulse width is one sampling clock period, even for signal conditions close to full modulation. This simplifies gate driver design and allows safe operation at theoretical full power. Nevertheless, 1-bit Σ-Δ modulation is not often used in Class D amplifiers (see Further Reading 4) because conventional 1-bit modulators are only stable to 50% modulation. At least 64x oversampling is also required to achieve adequate audio-band SNR, so typical output data rates are at least 1 MHz and power efficiency is limited.
More recently, self-oscillating amplifiers have been developed, such as the one described in Further Reading 5. These amplifiers always include a feedback loop, with the characteristics of the loop determining the switching frequency of the modulator, rather than an externally supplied clock. The high frequency energy is often flatter than with PWM. Excellent sound quality is achieved due to the feedback, but the loop is self-oscillating and therefore difficult to synchronize with any other switching circuits, or to connect to a digital audio source without first converting the digital signal to analog.
The full-bridge circuit (see Figure 3) can use “three-state” modulation to reduce differential EMI. In traditional differential operation, the output polarity of half-bridge A must be opposite to the output polarity of half-bridge B. There are only two differential operating states: output A high, output B low; output A low, output B high. However, there are two additional common-mode states, where the polarity of the two half-bridge outputs is the same (both high or both low). One of these two common-mode states can be combined with the differential state to produce three-state modulation, where the differential input to the LC filter can be positive, zero, or negative. The zero state can be used to represent low power levels, replacing the switching between positive and negative states in the two-state scheme. During the zero state, the differential action of the LC filter is very small, which actually increases common-mode EMI but reduces differential EMI. The differential advantage only applies to low power levels, because the positive and negative states must still be used to deliver high power to the speaker. The changing common-mode voltage levels in the three-state modulation scheme are a design challenge for closed-loop amplifiers.
Figure 7. PWM principle and example
EMI Treatment
The high-frequency components of the Class D amplifier output deserve careful consideration. If not properly understood and handled, these components can generate significant EMI and interfere with the operation of other equipment.
There are two types of EMI to consider: those radiated into space and those conducted through speakers and power lines. The Class D amplifier modulation scheme determines the baseline spectrum of conducted and radiated EMI components. However, there are some board-level design techniques that can be used to reduce the EMI emitted by a Class D amplifier, regardless of its baseline spectrum.
A useful rule is to minimize the area of loops carrying high-frequency currents, because the strength of EMI-related problems is related to the area of the loop and the proximity of the loop to other circuits. For example, the layout of the entire LC filter (including the speaker wiring) should be as tight as possible and kept close to the amplifier. The current drive and return traces should be grouped together to minimize the loop area (using twisted-pair wiring for the speaker helps). Another point to note is that when the gate capacitance of the output stage transistor is switched, a large transient charge is generated. Usually this charge comes from the energy storage capacitor, forming a current loop containing two capacitors. The EMI effect of transients in the loop can be reduced by minimizing the loop area, which means that the energy storage capacitor should be charged as close to the transistor as possible.
Sometimes it is helpful to insert RF chokes in series with the amplifier supplies . Proper placement of these confines high-frequency transient currents to a local loop close to the amplifier rather than conducting them long distances along the power supply lines.
If the gate drive non-overlap time is very long, the inductive current from the speaker or LC filter can forward bias the parasitic diode at the output stage transistor end. When the non-overlap time ends, the diode bias changes from forward to reverse. Before the diode completely turns off, a large reverse recovery current spike occurs, creating a troublesome source of EMI. EMI is minimized by keeping the non-overlap time very short (also recommended to minimize audio distortion). If the reverse recovery solution is still unacceptable, a Schottky diode can be used in parallel with the parasitic diode of the transistor to divert the current and prevent the parasitic diode from always turning on. This is helpful because the metal semiconductor junction of the Schottky diode is essentially immune to reverse recovery effects.
LC filters with toroidal inductor cores can minimize the effects of stray field power lines caused by amplifier currents. A good compromise between cost and EMI performance is to reduce radiation from low-cost drum cores through shielding, provided care is taken to ensure that this shielding acceptably degrades inductor linearity and speaker sound quality.
LC filter design
为了节省成本和PCB面积,大多数D类放大器的LC滤波器采用二阶低通设计。图3示出一个差分式二阶LC滤波器。扬声器用于减弱电路的固有谐振。尽管扬声器阻抗有时近似于简单的电阻,但实际阻抗比较复杂并且可能包括显著的无功分量。要获得最佳滤波器设计效果,设计工程师应当总是争取使用精确的扬声器模型。
A common filter design choice is to minimize the roll-off of the filter response to achieve the lowest bandwidth at the highest audio frequency required. If a roll-off of less than 1 dB is required for frequencies up to 20 kHz, a typical filter is required to have a 40 kHz Butterworth response (to achieve a maximally flat passband). For common speaker impedances and standard values of L and C, the following table gives nominal component values and their corresponding approximate Butterworth response:
If the design does not include speaker feedback, the speaker THD will be sensitive to the linearity of the LC filter components.
Inductor Design Considerations: Important factors in designing or selecting an inductor include the current rating and shape of the core, and the winding resistance.
Rated current: The rated current of the selected core should be greater than the maximum current of the expected amplifier. The reason is that if the current exceeds the rated current threshold and the current density is too high, many inductor cores will undergo magnetic saturation, resulting in a sharp decrease in inductance, which is not desirable.
An inductor is formed by winding wire around a core. If there are many turns of wire, the resistance related to the total wire length is important. Since this resistance is in series between the half-bridge and the speaker, it will dissipate some of the output power. If the resistance is too high, a thicker wire should be used or a core of another metal material should be selected that requires fewer turns of wire to provide the required inductance.
Finally, don't forget that the shape of the inductor used will also affect EMI, as mentioned above.
System Cost
What are the important factors that affect the overall cost of an audio system using Class D amplifiers, and how can we minimize the cost?
The active components of a Class D amplifier are the switching output stage and the modulator. The cost of building this circuit is roughly the same as an analog linear amplifier. The real tradeoffs to consider are the other components of the system.
The low power consumption of Class D amplifiers saves the cost (and PCB area) of heat dissipation devices, such as heat sinks or fans. Class D integrated circuit amplifiers can be packaged in smaller and lower-cost packages than analog linear amplifiers. When driving a digital audio source, analog linear amplifiers require a digital-to-analog converter ( DAC ) to convert the audio signal to an analog signal. This conversion is also required for Class D amplifiers that process analog inputs, but Class D amplifiers with digital inputs effectively integrate the DAC function.
On the other hand, the main cost disadvantage of Class D amplifiers is the LC filter. The components of the LC filter, especially the inductor, take up PCB area and increase cost. In high-power amplifiers, the overall system cost of Class D amplifiers is still competitive because the cost of the LC filter can be offset by the significant cost savings in heat sinks. But in low-cost, low-power applications, the cost of the inductor is high. In rare cases, such as low-cost amplifiers for cell phones, the cost of the amplifier IC may be lower than the total cost of the LC filter. Even ignoring cost considerations, the PCB area occupied by the LC filter is an issue in small applications.
To meet these concerns, the LC filter is sometimes eliminated entirely to achieve a filterless amplifier design. This saves cost and PCB area, although the benefits of a low-pass filter are lost. Without a filter, the increase in EMI and high-frequency power dissipation will be unacceptable unless the speaker is inductive and very close to the amplifier, the current loop area is minimized, and the power level is kept low. Although this design is often used in portable applications, such as cellular phones, it is not suitable for high-power systems, such as home audio.
Another approach is to minimize the number of LC filter components required for each audio channel. This can be achieved by using a single-ended half-bridge output stage, which requires half the number of inductors and capacitors as a differential full-bridge circuit. However, if the half-bridge output stage requires bipolar supplies, the cost associated with generating a negative supply may be too high unless the negative supply already serves some other purpose or the amplifier has enough audio channels to amortize the negative supply cost. Alternatively, the half-bridge can be powered from a single supply, but this reduces the output power and often requires the use of a large DC blocking capacitor.
Analog Devices Class D Amplifiers
All of the design issues just discussed can be reduced to a fairly demanding project. To save the design engineer time, Analog Devices offers a variety of Class D amplifiers IC1 that include programmable gain amplifiers, modulators, and power output stages. To simplify evaluation, Analog Devices provides demonstration boards for each type of amplifier. The PCB layout and bill of materials for these demonstration boards can serve as a practical reference design, helping customers quickly design proven, cost-effective audio systems without having to "reinvent the wheel" to solve the main design issues of Class D amplifiers.
For example, consider the AD1990, AD1992, AD1994, and AD1996 dual amplifier IC family, which are suitable for medium-power stereo or mono applications requiring two channels with outputs of 5, 10, 25, and 40 W per channel. Here are some of the features of these ICs:
The AD1994 Class D audio power amplifier contains two programmable gain amplifiers, two Σ-Δ modulators, and two power output stages to drive full H-bridge connected loads in home theater, automotive, and PC audio applications. It generates a switching waveform that can drive two 25 W stereo speakers, or a 50 W mono speaker, with 90% efficiency. Its single-ended input is applied to a programmable gain amplifier (PGA) with gain settings of 0, 6, 12, and 18 dB to handle low-level signals.
The AD1994 has integrated protection to prevent the output stage from being damaged by overheating, overcurrent, and inrush current. Due to its special timing control, soft start, and DC offset calibration, the click associated with mute is very small. Its main performance indicators include 0.001% THD, 105 dB dynamic range, greater than 60 dB PSR, and the use of switching output stage continuous time feedback and optimized output stage gate driver. Its 1 bit Σ-Δ modulator is especially enhanced for Class D applications to achieve 500 kHz average data frequency, with high loop gain for 90% modulation, and full modulation stability. The independent modulator method allows driving external large output power field effect tubes (FETs).
The AD1994 uses a 5 V power supply for the PGA, modulator, and digital logic, and an 8 V to 20 V high voltage power supply for the switch output stage. The associated reference design meets FCC Class B EMI standards. When driving a 6Ω load with 5 V and 12 V power supplies, its quiescent power consumption is 487 mW, the power consumption is 710 mW under 2×1 W output power conditions, and the power consumption in standby mode is 0.27 mW. The AD1994 is packaged in a 64-lead LFCSP and operates over a temperature range of –40°C to +85°C.
Previous article:Comparative Analysis of Inverter Three-Level "1" Type and "T" Type Circuits
Next article:Application of natural gas generators in communication base stations
Recommended ReadingLatest update time:2024-11-17 00:48
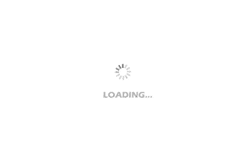
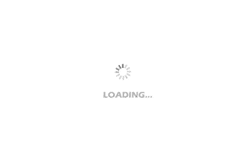
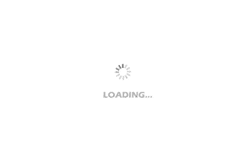
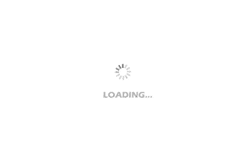
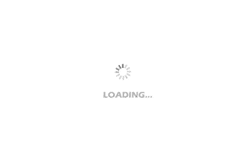
- Popular Resources
- Popular amplifiers
-
Operational Amplifier Practical Reference Handbook (Edited by Liu Changsheng, Zhao Mingying, Liu Xu, etc.)
-
A Complete Illustrated Guide to Operational Amplifier Applications (Written by Wang Zhenhong)
-
Design of isolated error amplifier chip for switching power supply_Zhang Rui
-
DAM medium wave transmitter high frequency power amplifier module test platform_Tian Tian
- MathWorks and NXP Collaborate to Launch Model-Based Design Toolbox for Battery Management Systems
- STMicroelectronics' advanced galvanically isolated gate driver STGAP3S provides flexible protection for IGBTs and SiC MOSFETs
- New diaphragm-free solid-state lithium battery technology is launched: the distance between the positive and negative electrodes is less than 0.000001 meters
- [“Source” Observe the Autumn Series] Application and testing of the next generation of semiconductor gallium oxide device photodetectors
- 采用自主设计封装,绝缘电阻显著提高!ROHM开发出更高电压xEV系统的SiC肖特基势垒二极管
- Will GaN replace SiC? PI's disruptive 1700V InnoMux2 is here to demonstrate
- From Isolation to the Third and a Half Generation: Understanding Naxinwei's Gate Driver IC in One Article
- The appeal of 48 V technology: importance, benefits and key factors in system-level applications
- Important breakthrough in recycling of used lithium-ion batteries
- Innolux's intelligent steer-by-wire solution makes cars smarter and safer
- 8051 MCU - Parity Check
- How to efficiently balance the sensitivity of tactile sensing interfaces
- What should I do if the servo motor shakes? What causes the servo motor to shake quickly?
- 【Brushless Motor】Analysis of three-phase BLDC motor and sharing of two popular development boards
- Midea Industrial Technology's subsidiaries Clou Electronics and Hekang New Energy jointly appeared at the Munich Battery Energy Storage Exhibition and Solar Energy Exhibition
- Guoxin Sichen | Application of ferroelectric memory PB85RS2MC in power battery management, with a capacity of 2M
- Analysis of common faults of frequency converter
- In a head-on competition with Qualcomm, what kind of cockpit products has Intel come up with?
- Dalian Rongke's all-vanadium liquid flow battery energy storage equipment industrialization project has entered the sprint stage before production
- Allegro MicroSystems Introduces Advanced Magnetic and Inductive Position Sensing Solutions at Electronica 2024
- Car key in the left hand, liveness detection radar in the right hand, UWB is imperative for cars!
- After a decade of rapid development, domestic CIS has entered the market
- Aegis Dagger Battery + Thor EM-i Super Hybrid, Geely New Energy has thrown out two "king bombs"
- A brief discussion on functional safety - fault, error, and failure
- In the smart car 2.0 cycle, these core industry chains are facing major opportunities!
- The United States and Japan are developing new batteries. CATL faces challenges? How should China's new energy battery industry respond?
- Murata launches high-precision 6-axis inertial sensor for automobiles
- Ford patents pre-charge alarm to help save costs and respond to emergencies
- New real-time microcontroller system from Texas Instruments enables smarter processing in automotive and industrial applications
- Discussion on the practical ability of silicone pads
- Shenzhen Electronic Hardware Engineer
- "Blink" on the "New Version of Bluesight AB32VG1 RISC-V Development Board"
- Share a book about Cortex M3 core
- msp430 MCU AD conversion
- Fudan Micro FM33LC046N Evaluation + ADC Collection Alarm
- MSP430FR235x-based pulse oximeter system solution
- CCS compilation error: Solution for missing header file
- LWIP uses UDP to send data continuously
- It was very popular in the past, and now it is back, how should this button position be designed?