Design of a Bandgap Reference Voltage Source for D/A Converter Circuit
[Copy link]
Abstract : This paper introduces the principle of bandgap reference voltage source and realizes a high-precision bandgap reference voltage source circuit. The effective temperature coefficient of this circuit is 6.1ppm/℃ in the temperature range of -20℃~100℃; when the power supply voltage changes from 1.6V to 2.0V, its power supply rejection ratio is 103.7dB. The reference voltage source plays an important role in the DAC circuit. The quality of its design directly affects the accuracy and stability of the DAC output. Temperature changes, fluctuations in power supply voltage and deviations in manufacturing processes will affect the characteristics of the reference voltage. This article makes a detailed analysis on how to design a reference voltage source with low temperature coefficient and high power supply voltage rejection ratio.
Considering the actual working environment of the DAC circuit, the power supply voltage range is 1.6V~2.0V, and the temperature range is -20℃~100℃. The design indicators of this bandgap reference voltage source are: 1. The output reference voltage is about 1.22V; 2. The power supply rejection ratio is 100dB; 3. The temperature coefficient of the reference voltage is less than 10ppm/℃. Principle of bandgap reference voltage source
The basic principle of the bandgap reference voltage source is: to use the base-emitter voltage V BE (with a negative temperature coefficient) of the bipolar transistor and their difference V BE (with a positive temperature coefficient) to compensate each other, so as to achieve the purpose of zero temperature coefficient of the circuit.
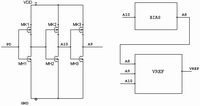 Figure 1 Schematic diagram of bandgap reference voltage generation Figure 2 Reference voltage source circuit
is shown in Figure 1. The function of its operational amplifier is to make the voltages at points X and Y equal when the circuit is in deep negative feedback. At this time, if R1=R2, then I1=I2, and it satisfies: V BE1 =V BE2 +I 2 R 3 (1) I 1 =I 2 =(1/R 3 )(V BE1 -V BE2 )=(1/R 3 )V T lnn (2) V OUT =V BE1 +I 1 R 1 =V BE1 +(R 1 /R 3 )V T lnn (3)
V OUT can be used as the reference voltage. From formula (3), it can be seen that the reference voltage is only related to the forward voltage drop of the PN junction, the ratio of the resistors, and the ratio of the emitter area of Q 1 and Q 2 , but has nothing to do with the input voltage. Therefore, it will have high precision in actual process manufacturing. The first term V BE1 has a negative temperature coefficient, which is about -2mV/℃ at room temperature; the second term V T has a positive temperature coefficient, which is about +0.085mV/℃ at room temperature. By setting a suitable operating point, the sum of the two terms can reach zero temperature coefficient at a certain temperature, thereby obtaining a reference voltage with good temperature characteristics. By properly selecting the values of R 1 , R 3 and n, an output voltage V OUT with zero temperature coefficient can be obtained . Circuit design
Based on the circuit principle shown in Figure 1, a reference voltage source circuit is designed, as shown in Figure 2. The circuit mainly consists of three parts: enable signal driving circuit, bias circuit, and bandgap reference voltage V REF generation circuit. Through the introduction of each part's structure and working principle, it can be seen that the circuit shown in Figure 2 can not only solve the problem of insufficient drive, but also flexibly adjust its temperature coefficient, and can achieve high power supply rejection ratio and low temperature coefficient performance.
Enable signal driving circuit
This circuit is composed of the three-stage inverter shown in Figure 2. PD is the enable signal of the circuit, and outputs A10 and A9 are used to enable control of BIAS and V REF
. The addition of the enable signal can reduce power consumption. When the external digital signal has not been sent to the conversion circuit, the PD enable signal puts the reference voltage circuit in standby state, thereby reducing power consumption: when the external digital signal is sent to the conversion circuit, the PD enable signal enables the reference voltage circuit to work.
In order to achieve a larger driving capability, the PD signal can be passed through an inverter composed of M k1 , M K2 , M K3 , M h1 , M h2 , and M h3 , as shown in Figure 2. The aspect ratio of the inverter tube increases step by step, and the driving capability increases step by step. Outputs A10 and A9 can effectively drive the enable tubes of BIAS and V REF , solving the problem of insufficient driving caused by long wiring in the layout or parasitic capacitance of the back-end circuit tube.
Bias circuit
The bias circuit is used to provide bias to the operational amplifier of the reference voltage circuit, as shown in Figure 3.
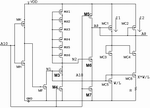 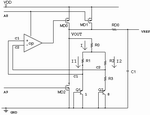 Figure 3 Bias circuit Figure 4 Reference voltage generation circuit
In the circuit of Figure 3, MK , MH , MF , M4 , M5 , and M7 are switch tubes, M3 , M6 , MS1 ~ MS6 areThe startup circuit is formed, and M C1 ~M C6 can establish a stable bias current.
When A 10 = 0, the switch tubes M K , M F , and M 5 are turned on, M H , M 4 , and M 7 are turned off, the bias circuit does not work, and A 8 = 1; when A 10 = 1, the switch tubes M H , M 4 , and M 7 are turned on, M K , M F , and M 5 are turned off, and the bias circuit works normally.
PMOS tubes MS1 ~ MS6 are resistor tubes. After power-on, M 6 is turned on, A 8 is pulled to a low level, and M C1 ~M C6 is turned on. The power supply current flows from M C1 through M C3 and M C5 to the ground, N 1 becomes a high level, M 3 tube is turned on, N 2 is pulled low, and M 6 tube is turned off. After a period of time, M C1 ~M C6 establish a stable bias current, and the startup circuit stops working.
M C1 ~M C6 and R can generate a current that is independent of the power supply . The current of the two branches of M C1 and M C2 is set by M C5 , M C6 , and R. In essence, I 1 is bootstrapped to I 2 , that is, I 1 =I 2. V GS5 = V GS6 + I 2 R (4)
(5) From formula (5), it can be seen that the current is independent of the power supply voltage, but is related to the aspect ratio of M C5 and M C6 and the value of the resistor. By adjusting these values, the required bias current can be easily obtained.
The reference voltage generation
circuit Figure 2 shows the V REF module, and its specific structure is shown in Figure 4.
This circuit filters out the high-frequency noise on the power line through R D0 and C 1 , making the reference voltage V REF more stable. Comparing Figure 4 with Figure 1, it can be seen that a resistor R 0
is added to the main structure of Figure 4. How to adjust the resistor to reduce the dependence of V REF on temperature can be explained by equations (6) to (11): I 1 R 1 =I 2 R 2 (6) V BE1 =V BE2 +I 2 R 3 (7) I=I 1 +I 2 (8) V OUT =V BE1 +I 1 R 1 +IR 0 (9) Substituting equations (6) to (8) into (9), we get: V OUT =V BE1 +AV T (10) (11) In equation (10), the first term V BE1 has a negative temperature coefficient, and the second term V T has a positive temperature coefficient. By properly selecting the values of R 0 , R 1 , R 2 , and R 3 and changing the size of A, the sum of the two terms can reach zero temperature coefficient at room temperature. Compared with equation (3), because the adjustable variable increases and the adjustment range becomes larger, V
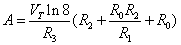 The temperature dependence of OUT is 0.
The design of the operational amplifier
in the reference voltage generation circuit requires the operational amplifier to have the largest gain possible and at the same time ensure that its phase margin is above 60 ° . The circuit is shown in Figure 5 .
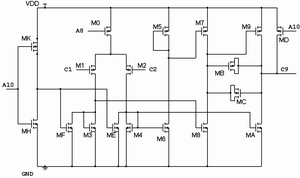 Figure 5 Operational amplifier circuit 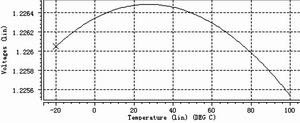 Figure 6 VREF temperature curve
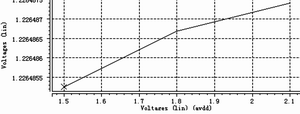 Figure 7 Reference voltage vs. power supply voltage curve In Figure 5, MK , MH , MF , ME , and MD are switch tubes controlled by enable signal A10. When A10 = 1, the operational amplifier works normally. MB and MC are capacitors made of MOS tubes and are used for margin compensation. Under the same area, the value of MOS capacitors can be much larger than that of polysilicon capacitors,
which greatly saves area. The amplifier adopts a two-stage push-pull output, which can obtain a very high gain and a large output swing. M0 mirrors the current in the bias circuit that is not related to the power supply to the M0 branch through A8 . The bias point and power consumption of the operational amplifier can be changed by adjusting the current in the bias circuit. Circuit Simulation Results
This design uses the 0.18 μ m? CSMC-HJ? N-well CMOS process model library and uses Hspice software to simulate the circuit.
Temperature characteristics
The power supply voltage is fixed at 1.8V, and the circuit is scanned from -20℃ to 100℃. The simulation results are shown
in Figure 6. As can be seen from Figure 6, the maximum and minimum values of V REF are 1.2265V and 1.2256V respectively. At 27℃, the reference voltage is 1.2265V. The temperature coefficient TC F of V REF can be measured by the following formula: (12)
As shown in Figure 6, the reference voltage changes with temperature, but the change is very small. From formula (12), it can be seen that TCF < 10ppm/℃, which meets the requirements of the DAC circuit for the reference voltage.
The
power supply voltage of the circuit is DC scanned, and the waveform obtained by Hspice simulation is shown in Figure 7.
Figure 7 is a coordinate enlargement diagram. It can be seen from the figure that when the power supply voltage changes from 1.6V to 2.0V, the reference voltage only changes from 1.2264855V to 1.2264875V.
The power supply rejection characteristics of the reference voltage can be measured by PSRR, and the PSRR is calculated as follows:
 =153300=103.7dB (13)
It can be seen that the reference voltage has very good suppression performance for the power supply and hardly changes with the power supply voltage.
|