Excerpted from eeworldonline, Jon Gabay
Today almost everyone has wireless service. Since the early days of analog wireless service and the introduction of digital 3G and 4G, most companies have relied on their wireless service providers and devices to stay connected to our fast-paced, real-time world. Now, engineers are looking into 5G connectivity to evaluate its suitability for their designs and applications.
5G is not the only protocol and standard, nor even the best one for many specific designs and future products. Many mature, feature-rich wireless protocols, devices, and development modules are readily available to quickly get your design up and running as quickly as possible.
While time to market is important, it is not the only constraint. In our wireless world, there is an entire ecosystem of devices and features that live in their own frequency bands, interacting with others in a lesser way. What standards and protocols best suit your needs depends on where you fall in the ecosystem. Let’s review.
What do you need?
The best isn’t necessarily the right fit. We all want 1ms latency and the 10Gbs data rates that 5G promises. But for our needs, that seems like shooting a fly with an anti-aircraft gun — making a mountain out of a molehill.
If a mobile robot surgeon performing life-saving surgery requires low latency and high-speed two-way video and audio streaming, as well as haptic feedback and a host of sensors and actuators, then 5G will be of great use here, but automating the light switches in your apartment does not.
Mobile designs typically have low power constraints but may also take advantage of widely deployed 3G, 4G, and 5G wireless networks. Fixed-location designs may harvest power locally or even harvest energy from the environment to enable long-distance wireless connections.
For example, a distributed array of sensors deployed in a fixed area cannot afford the cost burden of making each sensor 5G-enabled. First, the design costs, certification time, and the additional cost of monthly services required for each sensor before mass production will make it unfeasible. Instead, a lower-cost, established alternative wireless protocol can be used to allow each sensor to communicate with a single-point aggregator. Because the components, development kits, and open source software are likely to exist, design testing will need to be done. This can be a hub-point star architecture or a mesh network. This depends largely on the distances you need to traverse and the data rates.
Typically, large distributed sensor networks (such as environmental sensors, geological sensors, radioactivity sensors, etc.) will only transmit data for short periods of time from time to time. Even though the transmit power may be higher in the moment, the average data rate and power are lower than more compact, higher data-driven designs. Typically, latency should not be an issue for our environmental sensors either.
Furthermore, if you want to make the entire network globally accessible, you only need one aggregator or gateway. This significantly reduces operator costs. In this low-cost, low-data-rate, high-latency example, the aggregator can be 3G or 4G, and the performance is very good, because 3G and 4G are more widely deployed almost everywhere, so there is no need to use 5G equipment.
Back to Basics
For many companies, time to market is critical. Using newer technology is always riskier than using proven solutions. In this regard, more mature standards such as 3G and 4G provide designers with more options to adapt their designs to readily available parts and development environments.
When implementing multiple wireless communication links within a single device, other issues come into play. Frequency band overlap, shared antennas, metering power, beacons, and listening characteristics need to be considered.
We already have wireless multi-port communications 3G/4G, Wi-Fi and Bluetooth in our phones. Thankfully, ultra-high volume phone manufacturing has paved the way for more powerful, lower cost technology. But far fewer designers are designing phones than IoT devices.
By its very nature, IoT will add more connected devices to the globally accessible network.
We mentioned the vast array of environmental sensors and automated remote control technologies that we use in our lives, such as light switches, temperature and humidity sensors. We also have medical devices that can be worn or implanted in the body for treatment and exercise. Power plants, water treatment facilities, traffic lights, factories and self-driving cars, etc., all illustrate the range of demands we have on our design and communication infrastructure.
The most common choices are Wi-Fi and Bluetooth. Wi-Fi is heavily deployed and most facilities, commercial, industrial, and consumer locations offer Wi-Fi connectivity. For on-premises facilities, having multiple routers, bridges, gateways, and even a mesh topology is a great option, but the stadium problem is a different story.
Service degradation occurs when thousands of users are clustered in an area with limited bandwidth, channels, and sockets. This is known as the stadium effect. In a stadium, everyone is streaming the game to their friends on their phones. At some point, the available bandwidth is maxed out and service is disrupted. The same is true for 3G and 4G service near cell towers, which are already oversubscribed.
For IoT designs that must compete for time slices of bandwidth, alternative protocols may be more appropriate. For example, ZigBee has been around for many years, is supported by many device manufacturers, and provides open source code. It is a mesh network topology that can span large distances by storing and forwarding packets. It can coexist with 3G/4G/Wi-Fi and Bluetooth to create an independent network with fewer devices and required space. For example, security networks can benefit from this in-demand network.
There are also long-range subnets like Long Range LoRa that use the 915MHz band that excludes 3G/4G/5G, ZigBee, Bluetooth, and Wi-Fi entirely. This may be an ideal choice for widely distributed topologies such as marine and seismic sensors.
The LoRa long-range protocol can be used to create a separate wide-area network for sensors or IoT clusters that aggregate into a central 4G or 5G connection.
Benefits of the cloud
Although the cloud was originally used for storage and backup, it has evolved into a server for client applications. This means that applications and data are stored remotely, and our mobile devices are just terminals into the cloud window. Applications running in the cloud only need to refresh our remote devices.
This has both advantages and disadvantages. The advantage is that large data sets can be stored and retrieved when needed, and our devices can free up local memory. The data transfer from cloud storage to cloud processing happens completely in the background, is faster, and does not waste our time moving files around within our devices.
This approach relies on connectivity. If you don’t have a local copy of your applications and data, you’ll fail completely if the connection fails. However, this also opens the door to more complex applications such as self-driving cars, drones, agricultural equipment, and perhaps one day, airplanes.
Our handheld devices may not contain all the information about traffic, accidents, and detours, but the cloud does. This means that high-end processing can happen transparently in the background while tokenized commands and responses are sent between the cloud and the end device.
This could reduce overall data demand, especially with the low latency promised by 5G. It could also provide more data bandwidth for other critical infrastructure services, such as high-resolution surveillance cameras that are widely distributed across the urban landscape.
Overall, bandwidth and memory for remote handheld devices come at a cost. At some point, even handheld devices require a lot of fast data streams. Higher-resolution displays, faster refresh rates, and applications such as immersive virtual reality are multiplying the amount of data and the speed required. For example, a 2D flat screen might use 4K x 4K pixels; a 3D VR headset needs to store 360 degrees of data to react quickly enough to head movements.
So, what does 5G give us?
5G servers offer 1,000 times more bandwidth than 4G. That alone is astounding, especially given the data-intensive applications mentioned earlier. Here’s a major benefit: 5G can handle the potential traffic, and as the Internet of Things heats up, more connected devices will compete for channel space.
5G claims to be able to handle 10,000 times more traffic, which in the short term will eliminate the delays associated with oversubscription and provide a fabric for more connected devices as the Internet of Things grows. That’s 100 times the number of connected devices as 4G was able to connect.
5G's 10Gb/s maximum transmission speed is 10 times faster than 4G's 1Gb/s rate, but the difference in latency is eye-opening. 4G technology has a latency of 30 to 70 milliseconds. It may not seem like much, but it's sluggish if you're trying to practice with other music lovers remotely over the Internet. The variable delay time between all the players will make the result out of sync.
But 5G's promise of 1ms latency will allow you to enjoy music and watch football games online, and digital delays in voice conversations will no longer drive us crazy.
These performance improvements make it more likely that self-driving cars will be able to safely adapt to the environment in real time. Imagine if a 112.63km/h self-driving car could monitor and adjust its route every millisecond, you would feel extremely smooth. The same is true for drones, robots and automated factories.
There is also an as-yet-unproven claim that 5G devices will require 90% less power than comparable 4G devices. Only if 5G micro-base stations are spread all over the place will devices not need to use as much transmission energy to hit the base stations. Even so, this means that remote applications using energy harvesting (such as earthquake sensors) could run forever.
Product application cases
In fact, 4G isn't even deployed everywhere yet. In developing countries, there are no fixed lines and the entire communications infrastructure will be wireless. Designs using 4G technology are expanding, and many applications can make good use of 4G connections.
u-blox's mature products such as the SARA-R510M8S-00B connect wirelessly via LTE-M, and the SARA-R5 LTE-M and NB-IoT cellular series are now in mass production and certified by several major North American carriers for LTE-M. The SARA-R5 series is the first product to have a built-in UBX-R5 chipset and be certified by North American carriers. The UBX-R5 is u-blox's self-developed low-power wide-area (LPWA) chipset, which was certified by MNOs (mobile network operators) in early 2020 and is also the first IoT chipset to be certified by the Global Certification Forum (GCF). At 3.8V, it consumes 395ma when transmitting and is easily placed on a standard circuit board design.
The SARA-R5 series is suitable for LPWA IoT applications such as industrial automation, sensor applications, connected medical devices, smart meters, asset and vehicle tracking, and information and communication systems. The series provides powerful cloud connectivity while ensuring its security by design.
Although 4G base stations are still under construction, with the increase of equipment, equipment and demand, the transformation of existing base stations and 5G server base stations will increase.
Currently, 5G is used only as a short-distance backbone link between high-line-of-sight towers. As 5G chip components emerge, you don’t have to be a phone maker to get the parts, development systems and source code.
For example, ADI is producing several key parts for integrated 5G systems, such as the ADMV4801 RF transceiver and beamformer. Beamforming is an important component of 5G, and 5G servers and devices can position transmission targets into more powerful directional communication beams. The part operates in the 24GHz to 29.5GHz range and supports 16 transmitter channels and 16 receiver channels. Matched 50Ω input and output impedances make it easy to interface with standard antennas and RF components. It retains memory for beam positioning, which is useful for reconstructing communications in fixed point-to-point locations.
The ADMV1017 24GHz to 29.5GHz 5G up/down converter is part of a new portfolio of millimeter wave (mmWave) 5G solutions. Combined with it, it forms a viable, low-risk 5G embedded solution. Up/down modes include direct (from differential baseband) or single-ended IF to RF sideband upconversion. It has direct down converter, image rejection, or single-ended complex mode.
in conclusion
As equipment manufacturers and service providers deploy more towers and infrastructure, the 5G spectrum will be filled with traffic. With the steering of beams and the increase in energy in new frequency bands, we need to determine the safety of this technology for organic life. Although there is little non-commercially oriented research, this is a focused wavelength with a high probability of producing mutations.
Looking ahead, LPWAN technology is expected to serve as a subnet wireless partner as it coexists well with 5G. Unlicensed standards, such as 3GPP's Release 16, support 5G NR unlicensed bands and other options also exist.
Narrowband RF subsystems have been a promising option and have demonstrated the ability to handle 50,000 devices in an arranged cluster. Narrowband uses less power and can more easily penetrate walls and floors in those hard-to-reach places.
Stadiums, concert halls and large gathering places are expected to see private Wi-Fi-like networks within the 5G space. It can be seen that new partitions will emerge in the 5G space, providing higher reliability and less interference for critical tasks such as autonomous driving, drones, power plants and medical implants.
Clustered networks based on Wi-Fi, 6LoPAN, Bluetooth, ZigBee, etc. will also live in this ecosystem and will likely converge on the 5G domain at some point. The clever division of protocols and bands used and how they are used can determine the effectiveness of next-generation designs specifically for the IoT.
Previous article:5G and WiFi 6 speed comparison
Next article:The number of 5G devices has exceeded the 600 mark, and more than 150 5G networks have been launched worldwide
Recommended ReadingLatest update time:2024-11-25 13:28
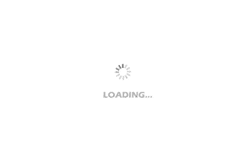
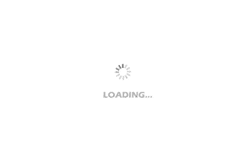
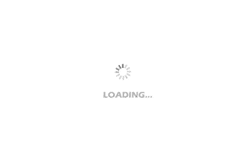
- Popular Resources
- Popular amplifiers
- Filling the domestic gap! China Mobile, Huawei and others jointly released the first GSE DPU chip
- Akamai Launches Cloud-Agnostic, Ready-to-Use App Platform
- IC China 2024 opens in Beijing: Intel shares insights to promote the implementation of intelligent computing applications
- Intel's Song Jiqiang: Collaborative innovation between cloud, edge and end to accelerate the application of intelligent computing
- Gartner: By 2027, 40% of AI data centers will be constrained by power shortages
- NVIDIA helps Google’s quantum AI accelerate processor design by simulating quantum device physics
- New breakthrough! Ultra-fast memory accelerates Intel Xeon 6-core processors
- Consolidating vRAN sites onto a single server helps operators reduce total cost of ownership
- Wi-Fi 8 specification is on the way: 2.4/5/6GHz triple-band operation
- Intel promotes AI with multi-dimensional efforts in technology, application, and ecology
- ChinaJoy Qualcomm Snapdragon Theme Pavilion takes you to experience the new changes in digital entertainment in the 5G era
- Infineon's latest generation IGBT technology platform enables precise control of speed and position
- Two test methods for LED lighting life
- Don't Let Lightning Induced Surges Scare You
- Application of brushless motor controller ML4425/4426
- Easy identification of LED power supply quality
- World's first integrated photovoltaic solar system completed in Israel
- Sliding window mean filter for avr microcontroller AD conversion
- What does call mean in the detailed explanation of ABB robot programming instructions?
- Automechanika Shanghai 2024 to feature a record number of concurrent events, bringing together industry wisdom and planning for future development
- Corporate Culture Sharing: How to cultivate scarce silicon IP professionals? SmartDV's journey of personal growth and teamwork
- Corporate Culture Sharing: How to cultivate scarce silicon IP professionals? SmartDV's journey of personal growth and teamwork
- NXP releases first ultra-wideband wireless battery management system solution
- NXP releases first ultra-wideband wireless battery management system solution
- Beijing Jiaotong University undergraduates explore Tektronix's advanced semiconductor open laboratory and experience the charm of cutting-edge high technology
- Beijing Jiaotong University undergraduates explore Tektronix's advanced semiconductor open laboratory and experience the charm of cutting-edge high technology
- New CEO: Dr. Torsten Derr will take over as CEO of SCHOTT AG on January 1, 2025
- How Edge AI Can Improve Everyday Experiences
- How Edge AI Can Improve Everyday Experiences
- RISC-V/ARM MCU IDE MounRiver Studio dual-core development project practice (CH32V103&CH32F103)
- "Playing board" + STM32F030Disco driver TM1628 control chip
- Positive feedback problem of Lm339 differential comparator
- Problems with drawing SOC-OCV curve (state of charge vs. open circuit voltage) while charging
- [Power supply production] Make a low-power linear power supply with waste materials
- [RISC-V MCU CH32V103 Review] 1. Unboxing and running
- Happy New Year!
- Structural Design of MSP430F148 Interrupt Stack
- [RVB2601 Creative Application Development] Embedded Development, I'm 10 Times Faster Than You -- RVB Dynamic Loading Model MBRE
- EEWORLD University Hall----2017 Toshiba PCIM Exhibition