Photovoltaic power generation systems use electronic components to convert solar energy into electrical energy. The inverter, as the core of the entire system, is usually divided into two categories: isolated and non-isolated. Combining the advantages of the two types of inverters is crucial to improving the efficiency, reliability, service life and reducing costs of the entire photovoltaic power generation system.
This article mainly introduces a high-frequency photovoltaic grid-connected inverter design using LLC resonant circuit, which combines the advantages of isolated and non-isolated types, reducing weight, reducing volume, reducing costs, and improving power quality and safety. In addition, since the use of LLC resonant circuit can realize soft switching of DC-DC level power devices, the switching loss of power devices can be greatly reduced, thus significantly improving the conversion efficiency of the entire system and the service life of the devices.
Photovoltaic grid-connected inverter structure and basic principles
System design structure
The structure of the photovoltaic grid-connected inverter using LLC isolation is shown in Figure 1. It includes a two-stage structure of a DC-DC direct current boost stage and a DC-AC inverter stage. The front stage is responsible for boosting and maximum power tracking the direct current transmitted from the solar cell array, and the rear stage is responsible for inverting the direct current transmitted from the front stage, and finally connecting to the grid after passing through the filter circuit.
How it works
Photovoltaic grid-connected inverters control the transmission of electric energy by regularly turning on and off power devices, which are controlled by pulse width modulation (PWM). The direct current generated by solar cells is first sent to the DC-DC circuit, and the DC-DC stage executes the maximum power point tracking (MPPT) algorithm to ensure that the solar cells always operate at the maximum power point.
After the maximum power point tracking control, the DC-DC circuit boosts the electric energy of the solar cell into a DC power suitable for the DC-AC stage, and then sends it to the DC-AC stage to convert the DC power into AC power. The controller tracks and calculates the grid voltage or current phase taken by the sampling circuit, and then adjusts the DC-DC stage power device switch to make the output current of the inverter have the same frequency and phase as the grid voltage, and finally transmits the electric energy to the grid through the output filter circuit or isolation transformer. The DC-DC stage input in this article is 200~300 V, outputs 400 V DC voltage, outputs 500 W, and the power factor is not less than 94% when fully loaded. The DC-AC stage input DC voltage is 400 V, the power level is 600 W, and the power factor is 1.
LLC Circuit Analysis
This article uses LLC resonant circuit to replace the power frequency transformer for isolation, which is different from the traditional photovoltaic grid-connected inverter and also its advantage. The traditional power frequency isolation transformer is large, bulky and costly. The use of LLC resonant circuit for isolation can greatly reduce the size of the inverter system and improve efficiency and power density. The LLC resonant circuit is based on the traditional series resonant circuit, and the transformer excitation inductance Lm is connected in series in the resonant circuit to form an LLC resonant circuit. Compared with the traditional series resonant circuit, the resonant frequency of the circuit is reduced due to the addition of a resonant inductor, and the zero voltage switching of the switch tube within the full load range can be achieved without the use of an additional auxiliary network; secondly, the transformer secondary rectifier diode can conditionally operate at zero voltage shutdown, reducing the loss caused by the reverse recovery of the diode; and it is suitable for working under a wide voltage input range. The higher the input voltage, the higher the efficiency. When the working point is optimal, a conversion efficiency of 97% can be obtained.
This article uses a half-bridge LLC series resonant circuit, as shown in Figure 2. The half-bridge LLC series resonant circuit includes input capacitors C1, C2, MOSFETs Q1, Q2, resonant inductor Lr, resonant capacitor Cr, transformer T1, output rectifier diodes D1 ~ D4 and output capacitor C3.
Due to the addition of a resonant inductor, the LLC resonant circuit has two resonant frequencies, one is the resonant frequency fr of the resonant inductor Lr and the resonant capacitor Cr, and the other is the resonant frequency fm of Lm plus Lr and Cr. The calculation formula is as follows:
In the series resonant circuit, the switch tube can only work in the ZVS state when the operating frequency fs is higher than fr. In the LLC circuit, the ZVS of the switch tube can be achieved as long as fs is higher than fm. The following is a simple analysis of its working process.
LLC circuit can be divided into four modes according to the switching frequency range. This article only discusses the working principle under the fr》fs》fm mode. The entire working process in one switching cycle is as follows. The working waveform is shown in Figure 3. PS1 and PS2 are the driving pulse waveforms of Q1 and Q2 respectively:
[t0 - t1] stage: At t0, the resonant current is negative, the Q1 body diode is turned on, and the voltage across Q1 is clamped at 0. At this time, Q1 is turned on at zero voltage. Energy flows from the positive pole of the power supply to the midpoint of C1 and C2, Lr and Cr resonate, and the resonant current ILr passes through the switch tube Q1 and gradually rises in a sinusoidal form. The current IT1 flowing through the primary side of the transformer is the difference between the resonant current ILr and the excitation current ILm. The polarity of the primary voltage of the transformer is positive at the top and negative at the bottom, and the polarity of the secondary side is also positive at the top and negative at the bottom. Therefore, D1 and D4 are naturally turned on, and the primary voltage of the transformer is clamped at nVo (n is the transformer ratio), and the excitation current rises linearly.
After half a cycle of resonance, Q1 is still in the on state. After half a cycle, the resonant current begins to decrease, and the excitation current continues to rise linearly. At t1, the resonant current is equal to the excitation current.
[t1 - t2] stage: At t1, the resonant current ILr is equal to the excitation current ILm, the transformer primary voltage is 0, the secondary voltage is also 0, the secondary rectifier diodes are all cut off, the primary no longer provides energy to the secondary, and the excitation inductance Lm begins to participate in the resonance. Since Lm is much larger than Lr, the LLC resonance period becomes significantly longer, so the resonant current remains basically unchanged. At t2, Q1 is turned off.
[t2 - t3] stage: At t2, Q1 is turned off, and Q2 is also in the off state, and the circuit enters the dead time. The resonant current ILr discharges the junction capacitance of Q2. When its voltage drops to 0, the body diode is turned on, the polarity of the primary winding of the transformer becomes negative at the top and positive at the bottom, the secondary rectifier diodes D2 and D3 are naturally turned on, and the voltage of the excitation inductor Lm is clamped by the output voltage and no longer participates in the resonance. The resonant current begins to decrease sinusoidally with a period of 2πLrCr, and the excitation current decreases linearly. At t3, Q2 is turned on with zero voltage.
[t3 - t4] stage : At t3, Q2 is turned on with zero voltage. Similar to the first stage, Lr and Cr resonate, the resonant current decreases in a sinusoidal form, and the excitation current decreases linearly. At t4, the resonant current is equal to the excitation current.
[t4 - t5] stage: From t4, the transformer primary voltage is 0, the secondary rectifier diodes are all cut off, the primary no longer provides energy to the secondary, the excitation inductor is no longer clamped by the output voltage, and begins to participate in resonance. The LLC resonant current remains basically unchanged.
[t5 - t6] stage: Similar to [t2 - t3] stage, the circuit enters the dead time, Q1 and Q2 are all turned off, and the resonant current ILr charges the junction capacitance of Q1. When its voltage is equal to the power supply voltage, the body diode is turned on, the polarity of the primary winding of the transformer is positive at the top and negative at the bottom, and the secondary rectifier diodes D1 and D4 are naturally turned on. The voltage of the excitation inductor Lm is clamped by the output voltage and no longer participates in the resonance.
The resonant current starts to increase sinusoidally with a period of 2πLrCr, and the excitation current increases linearly. At t6, Q1 is turned on with zero voltage, and the next cycle begins.
In the [t1 - t2] stage and the [t4 - t5] stage, assuming that the resonant current remains unchanged and is set to Im, the output voltage Uo can be expressed as:
In the formula: Ui is the input voltage; T is the switching period; Ts is the resonant period when Lr and Cr resonate. It can be seen from the formula that when T = Ts, that is, fr = fs, in this case, the [t1 - t2] stage and the [t4 - t5] stage will not exist, the resonant current is a pure sine wave, the output current of the secondary rectifier circuit is critically continuous, the root mean square value is the smallest, the switch tube conduction loss is the smallest, and the circuit efficiency is the highest [8]. Therefore, when the LLC circuit operates at the resonant frequency, the efficiency is the highest. The main function of the LLC circuit in this article is isolation. On the basis of ensuring isolation, the efficiency must be maximized. Therefore, in this article, the switching frequency of the switch tube is equal to the resonant frequency. Maximum power point tracking control strategy
Maximum Power Tracking Basic Principles
Solar cells are a nonlinear DC power source, and their output is greatly affected by the sunlight conditions and temperature. Under certain solar illumination and junction temperature conditions, when the terminal voltage (current) of the photovoltaic cell changes, its operating point will also change along the curve. However, there must be a point where the power output of the solar cell is the maximum. This point is called the maximum power point, and the technology for finding this maximum power point is called Maximum Power Point Tracking (MPPT).
In conventional linear system electrical equipment, in order to obtain maximum power, the load resistance needs to be equal to the internal resistance of the power supply. However, a solar cell is a nonlinear power source, and its internal resistance is constantly changing due to environmental influences. In order to match the load resistance and obtain maximum power, the load resistance value needs to be constantly adjusted. The equivalent resistance of the DC-DC converter is related to the working state of the switch tube, so its equivalent resistance can be changed by adjusting its duty cycle, so that its equivalent resistance value is always equal to the internal resistance of the solar cell, so that the solar cell can always work at the maximum power point.
This is the basic principle of maximum power point tracking for photovoltaic grid-connected inverters.
Maximum Power Tracking Algorithm
At present, the commonly used maximum power tracking algorithms mainly include constant voltage tracking method, perturbation observation method, conductance increment method, etc. Among them, the conductance increment method is favored for its excellent tracking performance. The following is a brief introduction to its working principle. Figure 4 is a solar cell characteristic curve. It can be seen from the figure that at the maximum power point, the slope of the power curve is 0, that is, the derivative of power P to voltage V is 0, so dPdU = 0, and because P = UI, so:
It can be seen from the above formula that when the change in output conductance is equal to the negative number of the output conductance, the solar cell operates at the maximum power point. The specific implementation method is: by detecting the output voltage and current of the solar cell, the change is calculated according to the values of voltage and current in the previous sampling period; then determine whether the change in voltage is zero. If it is zero, then determine whether the change in current is zero. If both are zero, it means that the impedance is consistent, then the reference voltage Vref remains unchanged, and the duty cycle remains unchanged. If the voltage change is zero and the current change is not zero, it means that the light intensity has changed, and the disturbance direction is determined according to the direction of the current change. When the voltage change is not zero, determine whether it meets the above formula. If it does, it means it is at the maximum power point. If the conductivity change is greater than the negative conductivity value, it means that the slope of the power curve is positive, and the power point is on the left side of the maximum power point. Vref needs to be increased, otherwise Vref needs to be reduced.
Conclusion
In view of the shortcomings of traditional photovoltaic grid-connected inverters using industrial frequency transformers for isolation, this paper proposes a photovoltaic grid-connected inverter design scheme using a half-bridge LLC series resonant circuit for isolation. This design scheme compares and analyzes the traditional transformer-isolated photovoltaic grid-connected inverter and the photovoltaic grid-connected inverter isolated by LLC resonant circuit. It can be seen that the half-bridge LLC series resonant circuit can realize the zero-voltage switching of the open tube and reduce the switching loss, thereby greatly improving the conversion efficiency of the inverter system. In addition, the LLC resonant circuit is small in size, light in weight, low in cost, easy to miniaturize and modularize, which is conducive to the widespread promotion and use of photovoltaic grid-connected inverters, thereby proving that the scheme is very practical.
Previous article:How to determine the control method of resonant single-phase full-bridge inverter?
Next article:Well-designed flyback switching power supply transformer
Recommended ReadingLatest update time:2024-11-16 14:48
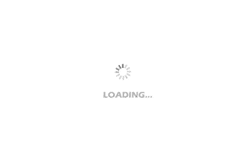
- Popular Resources
- Popular amplifiers
-
A high efficiency half-bridge LLC AC-DC converter design
-
Spread spectrum modulation suppresses conducted interference of LLC switching power supply_Geng Yanqing
-
Design of switching power supply based on dual-transformer LLC resonant converter
-
A review of switching power supply optimization methods based on LLC resonant converter
- MathWorks and NXP Collaborate to Launch Model-Based Design Toolbox for Battery Management Systems
- STMicroelectronics' advanced galvanically isolated gate driver STGAP3S provides flexible protection for IGBTs and SiC MOSFETs
- New diaphragm-free solid-state lithium battery technology is launched: the distance between the positive and negative electrodes is less than 0.000001 meters
- [“Source” Observe the Autumn Series] Application and testing of the next generation of semiconductor gallium oxide device photodetectors
- 采用自主设计封装,绝缘电阻显著提高!ROHM开发出更高电压xEV系统的SiC肖特基势垒二极管
- Will GaN replace SiC? PI's disruptive 1700V InnoMux2 is here to demonstrate
- From Isolation to the Third and a Half Generation: Understanding Naxinwei's Gate Driver IC in One Article
- The appeal of 48 V technology: importance, benefits and key factors in system-level applications
- Important breakthrough in recycling of used lithium-ion batteries
- Innolux's intelligent steer-by-wire solution makes cars smarter and safer
- 8051 MCU - Parity Check
- How to efficiently balance the sensitivity of tactile sensing interfaces
- What should I do if the servo motor shakes? What causes the servo motor to shake quickly?
- 【Brushless Motor】Analysis of three-phase BLDC motor and sharing of two popular development boards
- Midea Industrial Technology's subsidiaries Clou Electronics and Hekang New Energy jointly appeared at the Munich Battery Energy Storage Exhibition and Solar Energy Exhibition
- Guoxin Sichen | Application of ferroelectric memory PB85RS2MC in power battery management, with a capacity of 2M
- Analysis of common faults of frequency converter
- In a head-on competition with Qualcomm, what kind of cockpit products has Intel come up with?
- Dalian Rongke's all-vanadium liquid flow battery energy storage equipment industrialization project has entered the sprint stage before production
- Allegro MicroSystems Introduces Advanced Magnetic and Inductive Position Sensing Solutions at Electronica 2024
- Car key in the left hand, liveness detection radar in the right hand, UWB is imperative for cars!
- After a decade of rapid development, domestic CIS has entered the market
- Aegis Dagger Battery + Thor EM-i Super Hybrid, Geely New Energy has thrown out two "king bombs"
- A brief discussion on functional safety - fault, error, and failure
- In the smart car 2.0 cycle, these core industry chains are facing major opportunities!
- The United States and Japan are developing new batteries. CATL faces challenges? How should China's new energy battery industry respond?
- Murata launches high-precision 6-axis inertial sensor for automobiles
- Ford patents pre-charge alarm to help save costs and respond to emergencies
- New real-time microcontroller system from Texas Instruments enables smarter processing in automotive and industrial applications
- Please tell me about low noise broadband op amp
- How does MSP430FR6972 achieve the lowest power consumption for LCD driving?
- About the processing of C2000 bytes
- Why do we need to separate TX and RX? Let the data speak for itself!
- The meaning of communication IC pins
- The 2019 STM32 National Tour Seminar is about to start. Register now to win three gifts
- EEWORLD University Hall----Live Replay: Detailed Explanation of Maxim IO-Link Communication Protocol Design Solution
- LIS25BA Inclination Measurement Demonstration
- LED Art: LED Jewelry
- Is there anyone who chose the H topic? Let's exchange ideas.