As a special transformer, the leakage inductance transformer can not only transform the voltage, but also stabilize the voltage due to the existence of leakage inductance. This is because the magnetic flux generated when the primary voltage changes is not completely locked in the iron core to form the main magnetic flux, but a part of it is distributed between the coil and the air. When the primary voltage changes, the change of the secondary induced electromotive force will not be as drastic as that of an ideal transformer, which also plays a role in voltage stabilization.
Since the leakage inductance is distributed in the coil and the air, the traditional analysis method uses the path analysis method, which cannot calculate the exact distribution position and strength of the leakage inductance. For a long time, it can only be determined by experience. On the other hand, the traditional calculation method can only obtain macroscopic characteristics, but not the detailed internal structure of the transformer. In addition, the material of the iron core is generally nonlinear, which makes the calculation more difficult. Only the linear BH curve can be used instead of the solution, making the calculation inaccurate. In order to obtain accurate data of the transformer, it is necessary to rely on numerical calculation and computer technology.
ANSYS is a computational software based on the finite element method, which can be used to analyze many problems in the field of electromagnetic fields. It makes full use of the advantages of various computational methods and develops electromagnetic analysis modules suitable for different situations. Among them, the Emag module is mainly used for low-frequency electromagnetic analysis. Its main features are: nonlinear magnetic field analysis and field-circuit coupling analysis, which is very useful for calculating nonlinear materials, especially magnetic materials, and is mainly used in the fields of electric shock, transformers, electromagnetic switches, and induction heating.
1 Basic principles of transformer and leakage magnetic field
As shown in Figure 1, U1 is the primary coil voltage, N1 is the number of turns of the primary coil, U2 is the secondary coil voltage, N2 is the number of turns of the secondary coil. When a certain voltage is applied to the primary coil, an induced electromotive force will be obtained on the secondary coil according to the law of electromagnetic induction. In the absence of resistance, magnetic leakage and iron loss, the transformer is an ideal transformer, and the turns ratio of the primary coil and the secondary coil is equal to the ratio of the primary voltage to the secondary voltage, as shown in Figures 1 and 2.
As shown in Figure 2, if a sinusoidal AC voltage U1 is applied to both ends of the primary coil, an alternating current I1 will pass through the primary coil, thus exciting an alternating magnetic flux in the iron core. In order to facilitate the analysis of the problem, the total magnetic flux is divided into two equivalent parts of magnetic flux, one of which is closed along the iron core and interlinked with the primary and secondary coils at the same time, called mutual induction flux or main magnetic flux, represented by φ; the other part of the magnetic flux is mainly closed along the non-ferromagnetic material (such as air) and only interlinked with the primary line, called the primary coil leakage flux, represented by φ1, and another part that only interlinks with the secondary coil is called the secondary coil leakage flux, represented by φ2. The main magnetic flux accounts for the vast majority of the total magnetic flux, while the leakage flux accounts for only a small part (0.1% to 0.2%).
If we only rely on the leakage inductance between the air and the coil, we cannot meet the requirement of stable voltage of the leakage inductance transformer. Therefore, leakage magnetic punching sheets are artificially added between the primary and secondary coils to guide part of the magnetic field to pass through here, forming high leakage magnetic field.
2 Two-dimensional coupling simulation of leakage inductance transformer
ANSYS uses Maxwell's equations as the starting point for electromagnetic field analysis. In electromagnetic field calculations, Maxwell's equations are often simplified so that analytical solutions to electromagnetic fields can be obtained by using separation of variables method, Green's function method, etc. In actual engineering, ANSYS uses the finite element method to obtain numerical solutions based on the boundary conditions and initial conditions given in specific situations. The finite element method calculates unknown quantities (degrees of freedom) mainly as magnetic potential or flux, and the physical quantities of interest can be derived from these degrees of freedom. Depending on the unit type and unit options selected by the user, the degrees of freedom calculated by ANSYS are also different, which can be scalar magnetic potential, vector magnetic potential or boundary flux.
For transformers, it is necessary to study the parameters such as the magnetic field and secondary voltage generated by the external field that changes with time, so the two-dimensional vector potential method is used. The vector potential method has three degrees of freedom at each node, Ax, Ay, Az, which represent the magnetic vector potential degrees of freedom in the x, y, and z directions. In voltage feed or circuit coupling analysis, another three degrees of freedom are added to the magnetic vector potential degrees of freedom: potential (VO-LT), current (CURR), and electromotive force drop (EMF). The magnetic flux density can be calculated first from the vector magnetic potential. Its value is calculated by the unit shape function at the integration point. After obtaining B, the inductance of the coil can be obtained from the energy perspective, and then the inductance can be obtained based on the relationship between inductance and energy.
2.1 Pre-processing
By observing the structure of the transformer, we can find that the transformer is a symmetrical structure. Therefore, we can use its symmetry to simulate only a part of it to get the required result data.
According to the given size and material, the transformer model is established by selecting appropriate units, and the finite element model is established according to the actual size, and the mesh is divided and the degrees of freedom are coupled.
Since the magnetic field distribution in the core is mainly constrained by the excitation current under power frequency conditions and is basically not affected by eddy currents, it can be seen that the difference between the main magnetic flux of the core under load and no-load conditions is very small, so only the no-load condition can be considered. The two-dimensional finite element coupling model of the transformer is obtained (as shown in Figure 3).
This article mainly studies the magnetic field distribution of the transformer, especially the leakage flux. The leakage flux is mainly distributed between the air and the coil, so the air and coil parts should be drawn denser when dividing the grid. At the same time, the main magnetic flux is distributed in the iron core. In order to reflect the distribution and exact value of the main magnetic flux, the grid of the iron core also needs to be appropriately encrypted, see the partial diagram of the grid division. If only the direction problem is considered, a coarse grid can be used to shorten the calculation time.
2.2 Solution
Since the voltage frequency of the load is 50 Hz, the number of loading steps is calculated, and the time interval of each loading step is set to 1.25 ms. Each load step is divided into small steps with an interval of 0.25 ms. Due to the existence of coil inductance, it takes a while for the waveform to stabilize. Therefore, in order to obtain stable results, the loading time needs to be adjusted longer.
2.3 Post-processing
Using electromagnetic macros, we can obtain parameters such as the magnetic field lines and magnetic field strength vector at each load step or time point, as well as the induced voltage on the secondary coil.
When an alternating voltage is applied to the primary coil, according to Maxwell's equations, the changing electric field generates a changing magnetic field, which passes through the coil to form a closed magnetic field and spreads around the coil. Due to the constraints of the iron core, the magnetic field winds around the iron core to form a closed magnetic field, also known as the main magnetic flux.
Figures 4 and 5 show the simulated magnetic field distribution, from which the direction and size of the magnetic lines of force can be clearly observed. Through observation, it can be seen that the magnetic field distribution in the core is relatively uniform. Because it is a 1/2 model, the magnetic field in the middle of the core is more concentrated, so the magnetic induction intensity is relatively larger than the value at the edge of the core. The magnetic field intensity in the core is between 0.85 and 1.6 T, which is within the theoretical calculation range, thus proving the correctness of the model and calculation method.
Since there is no transformer without resistance and iron loss, transformers are not ideal and will have losses, which makes the voltage on the primary and secondary coils not completely conform to the voltage balance formula. When simulating, a 220 V power frequency voltage is added to the primary coil of the transformer. The simulation results show that the secondary voltage is about 2240 V (see Figure 6), which is lower than the secondary voltage of an ideal transformer and conforms to the characteristics of an actual transformer. Compared with the experimental test results, the error is within 3%, proving that the assumptions and methods of the simulation are correct.
If you look closely at Figure 5, you will find that there is a very narrow magnetic field channel between the primary and secondary coils (this is the magnetic flux guided by the leakage magnetic sheet), so that part of the magnetic field passes through here, forming leakage magnetic field. The existence of leakage magnetic field can be clearly observed through the three-dimensional model. When the leakage magnetic sheet grid is further subdivided and the actual BH curve is added (as shown in Figure 7), it is found that the leakage magnetic field increases from the original 0.1% to 1%, as shown in Figure 8.
7 represents the magnetic field intensity H, the unit is A/M, and the ordinate represents the magnetic induction intensity, the unit is T.
Comparing Figure 5 and Figure 8, it can be found that the magnetic field strength in the core has become smaller. This is because after adding the BH curve, it reaches saturation at around B=1.65 T (as shown in Figure 7), which inhibits the increase of the magnetic field in the core, making the magnetic field of the core not as strong as the magnetic field strength when the linear μ is used. It is also due to the inhibitory effect of BH that part of the magnetic field is diverted to the leakage magnetic punching sheet, forming a large leakage inductance.
By adjusting the voltage of the primary coil, the secondary voltage can also change. However, this phenomenon is not obvious in the leakage inductance transformer. When the primary voltage changes by 10% under the rated voltage, the change of the secondary voltage does not exceed 3% of the rated secondary voltage. This is because the magnetic field generated by the primary coil is not completely locked in the iron core to form the main magnetic flux, but a part of it leaks out. It is consistent with the leakage inductance effect of the actual leakage inductance transformer.
As shown in Figure 9, the horizontal axis represents the primary voltage of the leakage inductance transformer, and the vertical axis represents the secondary voltage, with the unit of V. As can be seen from Figure 9, the secondary voltage change curves of the ideal transformer and the leakage inductance transformer are consistent with the voltage change curve of the primary coil, but the secondary voltage of the ideal transformer is larger than the secondary voltage of the leakage inductance, and the increase is larger, that is, when the primary voltage changes, the secondary voltage of the ideal transformer changes more dramatically than the secondary voltage of the leakage inductance transformer. This is because the ideal transformer does not consider the loss of coil impedance, especially the influence of leakage inductance, so the secondary voltage changes dramatically. Figure 9 also proves the voltage stabilizing effect of leakage inductance from the side.
3 Conclusion
The transformer was simulated in two dimensions, and the voltage, current, and magnetic field distribution that were consistent with the actual situation were obtained, proving the correctness of the simulation modeling and calculation methods. The magnetic field distribution inside the transformer was obtained, especially the main magnetic flux in the iron core and the leakage magnetic flux distributed around the iron core. The existence of leakage inductance and its role in stabilizing voltage were confirmed. With the help of simulation software, the visualization of the magnetic field inside the transformer was realized, which provided a basis for the design of the transformer, saved design costs, and shortened the design cycle.
Previous article:Eight-Channel Data Acquisition System Using a Single ADC Driver
Next article:Reactive power compensation methods and some existing problems in power system
Recommended ReadingLatest update time:2024-11-16 21:55
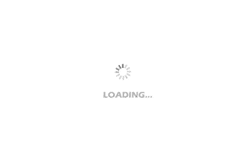
- Popular Resources
- Popular amplifiers
- MathWorks and NXP Collaborate to Launch Model-Based Design Toolbox for Battery Management Systems
- STMicroelectronics' advanced galvanically isolated gate driver STGAP3S provides flexible protection for IGBTs and SiC MOSFETs
- New diaphragm-free solid-state lithium battery technology is launched: the distance between the positive and negative electrodes is less than 0.000001 meters
- [“Source” Observe the Autumn Series] Application and testing of the next generation of semiconductor gallium oxide device photodetectors
- 采用自主设计封装,绝缘电阻显著提高!ROHM开发出更高电压xEV系统的SiC肖特基势垒二极管
- Will GaN replace SiC? PI's disruptive 1700V InnoMux2 is here to demonstrate
- From Isolation to the Third and a Half Generation: Understanding Naxinwei's Gate Driver IC in One Article
- The appeal of 48 V technology: importance, benefits and key factors in system-level applications
- Important breakthrough in recycling of used lithium-ion batteries
- Innolux's intelligent steer-by-wire solution makes cars smarter and safer
- 8051 MCU - Parity Check
- How to efficiently balance the sensitivity of tactile sensing interfaces
- What should I do if the servo motor shakes? What causes the servo motor to shake quickly?
- 【Brushless Motor】Analysis of three-phase BLDC motor and sharing of two popular development boards
- Midea Industrial Technology's subsidiaries Clou Electronics and Hekang New Energy jointly appeared at the Munich Battery Energy Storage Exhibition and Solar Energy Exhibition
- Guoxin Sichen | Application of ferroelectric memory PB85RS2MC in power battery management, with a capacity of 2M
- Analysis of common faults of frequency converter
- In a head-on competition with Qualcomm, what kind of cockpit products has Intel come up with?
- Dalian Rongke's all-vanadium liquid flow battery energy storage equipment industrialization project has entered the sprint stage before production
- Allegro MicroSystems Introduces Advanced Magnetic and Inductive Position Sensing Solutions at Electronica 2024
- Car key in the left hand, liveness detection radar in the right hand, UWB is imperative for cars!
- After a decade of rapid development, domestic CIS has entered the market
- Aegis Dagger Battery + Thor EM-i Super Hybrid, Geely New Energy has thrown out two "king bombs"
- A brief discussion on functional safety - fault, error, and failure
- In the smart car 2.0 cycle, these core industry chains are facing major opportunities!
- The United States and Japan are developing new batteries. CATL faces challenges? How should China's new energy battery industry respond?
- Murata launches high-precision 6-axis inertial sensor for automobiles
- Ford patents pre-charge alarm to help save costs and respond to emergencies
- New real-time microcontroller system from Texas Instruments enables smarter processing in automotive and industrial applications
- [Qinheng Trial] 2. Preparation for CH549EVT Operation
- A brief discussion on SSI interface technology and its application in audio processing
- PCB source file: adapter board for LIS25BA bone vibration sensor, thanks to ST engineers~
- Share BLE connection parameters and their setting points
- Photodiode Problems
- Interesting animated tutorial "Principles of Dialogue Communication"
- What interesting skills do Chinese people have? What about engineers?
- Design principles of fuel gauge circuit based on BQ40z80
- DSP28335 usage issues
- [Project source code] Using math library in Linux application reports undefined reference to `sin', etc.