Today, traditional analog oscilloscopes have gradually faded out of people's sight, and digital oscilloscopes have almost replaced analog oscilloscopes and become the most commonly used instrument for circuit debugging in the hands of hardware engineers. Do you think that the oscilloscope provides all the information of the measured signal? In fact, the oscilloscope is in a no-signal state most of the time and cannot detect the signal. This period of time when the signal is lost is usually called dead time.
What is dead time?
To understand the source of dead time, you need to have a basic understanding of the structure of a digital oscilloscope. The typical block diagram of a digital oscilloscope is shown in Figure 1 and Figure 2.
Figure 1: Block diagram of a traditional digital oscilloscope.
Figure 2: Block diagram of the RTO series oscilloscopes from R&S.
The measured signal enters the oscilloscope through the input channel and is conditioned by the attenuators and amplifiers in the vertical system. The analog-to-digital converter (ADC) samples the signal at fixed time intervals and converts each signal amplitude into a discrete digital value called a "sample point". The acquisition module then performs processing functions, such as sample extraction, and the default is generally sampling mode. The output data is stored as sample points in the acquisition memory. The number of stored sample points can be set by the user through the record length.
These sample points can be further post-processed according to user needs. Post-processing tasks include arithmetic functions (such as averaging), mathematical operations (such as FIR filtering), automatic measurements (such as rise time or fall time), and analysis functions (such as histogram or mask testing). Other post-processing tasks include protocol decoding, jitter analysis, and vector signal analysis.
For digital oscilloscopes, there is essentially no limit to the processing steps that can be performed on the waveform samples. These post-processing functions are either performed using software through the instrument's main processing routine, or using dedicated ASIC or FPGA hardware, depending on the oscilloscope's architecture. The final results are then presented to the user through the oscilloscope's display.
From Figures 1 and 2, we can see the difference in signal processing between the R&S RTO series oscilloscope and traditional digital oscilloscopes. It uses the specially developed ASIC chip RTC and FPGA to implement waveform sample post-processing, such as channel calibration, sample extraction, digital filtering, math, histogram measurement, template test, FFT, automatic measurement, protocol decoding, etc., which greatly reduces the workload of the main processor. At the same time, the analog trigger circuit is replaced by a digital trigger in the RTO chip, eliminating the trigger jitter caused by the analog trigger circuit. In order to reduce this part of the jitter, traditional mid-to-high-end oscilloscopes require a lot of DSP post-processing. Innovation in hardware structure has greatly shortened the time spent on waveform sample post-processing of the RTO oscilloscope.
The period from signal sampling to waveform sample processing and display is called capture period. The oscilloscope can capture the next new waveform only after the previous capture period ends. Therefore, the digital oscilloscope uses most of the capture period to post-process the waveform samples. During this process, the oscilloscope is in a no-signal state and cannot continue to monitor the measured signal. Fundamentally speaking, dead time is the time required for the digital oscilloscope to post-process the waveform samples.
Relationship between dead time, capture cycle and waveform capture rate
Figure 3 shows a schematic diagram of a waveform capture cycle. The capture cycle consists of an effective capture time and a dead time period. During the effective capture time, the oscilloscope captures the number of waveform samples set by the user and writes them into the acquisition memory. The capture dead time consists of a fixed time and a variable time. The fixed time depends on the architecture of each instrument. The variable time depends on the time required for processing, which is directly related to the set number of captured samples (record length), horizontal scale, sampling rate, and selected post-processing functions (such as interpolation, math functions, measurements, and analysis). The ratio of dead time to capture period The dead time ratio is also an important characteristic of the oscilloscope. The reciprocal of the capture period is the waveform capture rate.
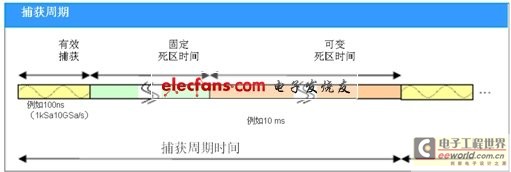
Figure 3: One capture cycle of a digital oscilloscope.
For example, if the effective capture time is 100ns (the number of samples is 1k, and the sampling rate is 10G), and the dead time is 10ms, then the time used for the entire capture cycle is 10.0001ms. The resulting dead time ratio is 99.999%, and the waveform capture rate is less than 100 waveforms per second. Currently, most oscilloscopes on the market have a waveform capture rate of several hundred times under conventional measurement mode. R&S's latest RTO series oscilloscopes can achieve a waveform capture rate of up to 1,000,000 times under the same conditions, and the dead time ratio can be reduced to less than 90%, which is much higher than other oscilloscopes. Some oscilloscopes with a bandwidth of ≤1G can achieve a waveform capture rate of 50,000 times/second at their highest sampling rate, and their dead time ratio is also as high as 99.5% or more. [page]
Effects of Dead Time and Waveform Capture Rate on Measurement Results
Many engineers may have encountered such a situation during hardware debugging: in the later stage of debugging, the welding of the main components of the circuit board is basically completed. During the functional verification process, it is found that the system will fail soon after running, but the key clock and enable signals are checked through the oscilloscope. There is "no problem", and the cause of the failure is finally determined to be software, and then the code is checked line by line to optimize the software. Now we have a clear understanding of the dead time of the oscilloscope. Another possibility for the above situation is that the oscilloscope missed the occasional signal that caused the system failure. Figure 4 can illustrate this problem very vividly:
Figure 4: Oscilloscope dead time causes loss of critical infrequent signals.
Due to the existence of the oscilloscope dead time, the oscilloscope may miss key abnormal signals and display a deceptive result to the user, ultimately misleading the user's judgment, greatly prolonging the debugging time and reducing debugging efficiency.
According to Equation 1, if the waveform capture time (that is, number of samples × resolution, or 10 × horizontal scale), waveform capture rate, and signal event occurrence rate (such as the repetition rate of a pulse interference) are all determined, increasing the measurement time will increase the probability of capturing and displaying the signal event:
Formula 1:
P: The probability of capturing an occasional repetitive signal event [in %]
GlitchRate: Signal failure frequency (e.g., repetitive pulse interference) [unit: 1/s]
T: effective capture time or waveform display time (record length/sampling rate, or record length × resolution, or 10 × time range/division) [unit: s]
AcqRate: waveform capture rate [unit is wfms/s]
Tmeasure: measurement time [unit: s]
If the probability is known, the time required to capture the sporadic signal can be calculated by transforming Formula 1:
Formula 2:
Assume that a signal has an anomaly that repeats 10 times per second. The signal itself is displayed as data on an oscilloscope with a horizontal scale of 10ns/div. If the display used has 10 horizontal divisions, an effective capture time of 100ns can be calculated. In order to ensure a high confidence level in capturing the desired signal event, a probability of 99.9% is used. Now, the required test time depends on the waveform capture rate of the oscilloscope. The following table summarizes the required test time corresponding to several different waveform capture rates.
Table 1: Time required to capture repetitive abnormal signals with a probability of 99.9% (T=100ns, GlitchRate=10/s).
Although the dead time ratio of R&S RTO series oscilloscopes under this condition is still close to 90%, compared with other oscilloscopes with a dead time ratio of more than 99.5%, its ability to detect occasional abnormal signals has increased by orders of magnitude, which can help engineers greatly improve debugging efficiency. Let me ask: How many engineers can watch each signal on the oscilloscope for more than 7 seconds?
As mentioned earlier, the waveform capture rate is related to the settings of the horizontal scale, record length, and sampling rate. In actual measurements, how to find a balance among these parameter settings based on the actual measured signal, view the waveform with the highest capture probability, and improve debugging efficiency is an issue that engineers need to consider when using digital oscilloscopes. This part will be discussed specifically in later articles.
Previous article:Basic Principles of Resistor Current Sensing
Next article:A typical differential amplifier circuit design and test
- Popular Resources
- Popular amplifiers
- Keysight Technologies Helps Samsung Electronics Successfully Validate FiRa® 2.0 Safe Distance Measurement Test Case
- From probes to power supplies, Tektronix is leading the way in comprehensive innovation in power electronics testing
- Seizing the Opportunities in the Chinese Application Market: NI's Challenges and Answers
- Tektronix Launches Breakthrough Power Measurement Tools to Accelerate Innovation as Global Electrification Accelerates
- Not all oscilloscopes are created equal: Why ADCs and low noise floor matter
- Enable TekHSI high-speed interface function to accelerate the remote transmission of waveform data
- How to measure the quality of soft start thyristor
- How to use a multimeter to judge whether a soft starter is good or bad
- What are the advantages and disadvantages of non-contact temperature sensors?
- Innolux's intelligent steer-by-wire solution makes cars smarter and safer
- 8051 MCU - Parity Check
- How to efficiently balance the sensitivity of tactile sensing interfaces
- What should I do if the servo motor shakes? What causes the servo motor to shake quickly?
- 【Brushless Motor】Analysis of three-phase BLDC motor and sharing of two popular development boards
- Midea Industrial Technology's subsidiaries Clou Electronics and Hekang New Energy jointly appeared at the Munich Battery Energy Storage Exhibition and Solar Energy Exhibition
- Guoxin Sichen | Application of ferroelectric memory PB85RS2MC in power battery management, with a capacity of 2M
- Analysis of common faults of frequency converter
- In a head-on competition with Qualcomm, what kind of cockpit products has Intel come up with?
- Dalian Rongke's all-vanadium liquid flow battery energy storage equipment industrialization project has entered the sprint stage before production
- Allegro MicroSystems Introduces Advanced Magnetic and Inductive Position Sensing Solutions at Electronica 2024
- Car key in the left hand, liveness detection radar in the right hand, UWB is imperative for cars!
- After a decade of rapid development, domestic CIS has entered the market
- Aegis Dagger Battery + Thor EM-i Super Hybrid, Geely New Energy has thrown out two "king bombs"
- A brief discussion on functional safety - fault, error, and failure
- In the smart car 2.0 cycle, these core industry chains are facing major opportunities!
- The United States and Japan are developing new batteries. CATL faces challenges? How should China's new energy battery industry respond?
- Murata launches high-precision 6-axis inertial sensor for automobiles
- Ford patents pre-charge alarm to help save costs and respond to emergencies
- New real-time microcontroller system from Texas Instruments enables smarter processing in automotive and industrial applications
- Sharing a carefully adjusted hysteresis comparator
- The simulation result of LC parallel resonance frequency is inconsistent with the calculation. Is it because the component model is not selected correctly?
- Using Bluetooth module for fast IoT circuit design
- Using the Ginkgo USB-I2C adapter and the BMP180/BMP085 sensor to implement an atmospheric pressure detector
- Disassembling the x990
- Greenhouse automatic spraying system ---- H743 water lamp
- Design of wireless serial port hub using ARM microprocessor and ZigBee module
- [TI recommended course] #Live replay: TI mmWave millimeter wave radar application in automobiles#
- 1602 display dht11 temperature and humidity
- CircuitPython 6.1.0 released