introduction
Limited bandwidth and the increasing demand for new wireless services have paved the way for the adoption of new technologies in the communications field. These non-traditional technologies have effectively increased data capacity. One of these newly adopted technologies is the multiple-input, multiple-output (MIMO) system architecture using multiple antenna designs. MIMO uses spatial diversity technology between transmit and receive antennas - multiple signal paths caused by signal fading and multipath environments - to increase data throughput without additional bandwidth. However, compared with traditional single-stream architecture MIMO, the system complexity has increased a lot, bringing greater testing challenges and requiring unique equipment and test methods.
This article introduces the different types of MIMO measurements, including the damage caused by noise and interference to the channel, and provides some picture examples to help everyone understand the measurement results.
High data throughput is a basic requirement for recent wireless communication standards, all of which involve MIMO, including IEEE 802.11n WLAN, IEEE 802.16e Mobile WiMAX Wave 2, and 3GPP Long Term Evolution (LTE). These new systems combine MIMO with OFDM or OFDMA (Orthogonal Frequency Division Multiple Access) to increase data throughput without increasing channel bandwidth.
Comparison of SISO and MIMO
In a traditional single-input, single-output (SISO) communication system (as shown in Figure 1a), for example, a traditional IEEE 802.11a/b/g wireless local area network (WLAN) system, a wireless link uses a single transmitter and a single receiver. Multiple antennas may be used at each end of the communication link, but only one set of antennas is used at the same time, and only one carrier transmits a single stream of data. In an ideal communication channel, the wireless signal is transmitted from the transmitter to the receiver through only a single path, but obstacles (such as buildings and various terrains) and movement in the wireless channel produce multipath effects, so the receiver will receive multiple signals. The reflected signal will be affected by attenuation and delay due to its longer propagation path than the directly transmitted signal. Because of the different transmission paths, the phases of these reflected signals are also different. Therefore, it is difficult to reconstruct the signal at the receiver, which will cause fluctuations in the received signal strength. Strong multipath effects will reduce throughput or cause data loss.
Figure 1. The traditional SISO wireless signal chain (a) uses a pair of antennas to transmit and receive at the same time, while the MIMO system (b) uses multiple signals and multiple antennas at the same time.
Because OFDM is often combined with MIMO to enhance data throughput in a given communication channel, it is important to understand OFDM before discussing the concept of MIMO. For example, OFDM is used in IEEE 802.11g (Wi-Fi) and IEEE 802.16e WiMAX systems. On the basis of MIMO, OFDM can be used to further improve data throughput without increasing bandwidth or changing the modulation order - such as changing from 16QAM to 64QAM system.
Wireless signals modulated with OFDM are essentially composed of a series of mutually orthogonal subcarriers, which form the best isolation between each other. Therefore, when a modulated subcarrier is at maximum power, its adjacent modulated subcarrier is just at the zero crossing point or the minimum power point, and some subcarriers are used as protection bands to achieve isolation and prevent adjacent channel interference. In order to enhance robustness, OFDM adopted by many communication standards adopts a small attenuation interval to allow multi-path signal components to decay over time, so that these signals will not interfere with the transmission symbol received by the next receiver.
By using inverse Fourier transform to perform digital signal processing on OFDM subcarriers, they can be combined into one signal stream for transmission and the original signal can be restored. Because the relative phase and frequency relationship of the multi-stream signals are retained, these signal streams can be transmitted in parallel on a single channel, so it is possible to increase data throughput without increasing bandwidth.
Compared with SISO communication systems, MIMO systems (Figure 1b) use multiple wireless signals and multiple antennas at the same time, and multiple data streams are transmitted on the same communication channel. These multiple data streams are coordinated by the media access control (MAC) layer at both ends of the communication link. MIMO systems do not require symmetrical arrangement of antennas, for example, two transmitters must be equipped with two receivers (2×2) or four transmitters must be equipped with four receivers (4×4), and can be "unbalanced" configurations, such as a 4×3 configuration with four transmitters and three receivers.
To increase the data throughput of a SISO system, more complex modulation methods, increased bandwidth, or a combination of both are required. The simplest way to double the throughput of a SISO system is to double the bandwidth. To increase the throughput of a MIMO system, the number of transmitters, receivers, and corresponding antennas needs to be increased. By using multiple antennas and spatial multiplexing of the signal propagation path, a MIMO system can increase throughput by about 3.5 times without increasing the channel bandwidth.
MIMO systems increase data throughput by taking advantage of changes in the received signal, which is viewed as a simultaneous equation of the unknown signal (the transmitted symbols). The diversity of the multiple signal paths makes these simultaneous equations easier to solve and increases throughput.
How does the channel capacity of SISO compare to that of MIMO systems? Shannon’s law states that the channel throughput of a SISO communication system is
C=BLog2(1+S/N)
Where: C is the channel capacity (in b/s), B is the channel bandwidth (in Hz), S is the total signal power in the bandwidth (in W or V2), and N is the total noise power in the bandwidth (in W or V2). When this formula is used for MIMO applications:
C = ABlog2(1+S/N)
Where: A is the number of transmitting antennas.
This equation points out the direct relationship between the number of transmit antennas and channel capacity in a MIMO system. A MIMO system uses spatial multiplexing technology to transmit multiple data streams using multiple antennas on the same physical channel. The data streams are sent on multiple transmitters without changing the symbol rate. By adding more transmitters and transmit antennas, the system throughput is improved without changing the bandwidth. [page]
Modeling a MIMO system must take into account the number of multiple data streams, including direct and reflected signals reaching the receiver. According to the traditional method, the transmitters are represented as Tx1, Tx2, ..., Txn, and the receivers are represented as Rx1, Rx2, ..., Rxn. A MIMO communication system can be represented by a matrix signal vector hxy, where x represents the number of transmitters and y represents the number of receivers. For example, h21 represents two transmitters and one receiver, while h22 represents two transmitters and two receivers (as shown in Figure 2). In this way, a MIMO channel can be modeled as follows:
y=H*x+n
Where: y is the received signal vector, H is the channel matrix (hxy signal element), x is the transmitted signal vector, and n is the noise vector.
Figure 2 The wireless channel in a MIMO system can be represented by a series of different vectors
The effects of different channels on the received signal, such as attenuation and multipath effects, can be corrected by the same algebraic equation, the relationship is
Rx=H*Tx+n
Where: Rx represents the Rx1, Rx2, ..., Rxn matrices of the receiving antennas, and Tx represents the Tx1, Tx2, ..., Txn matrices of the transmitting antennas. For a 2×2 MIMO system, the relationship is as shown in the matrix of Figure 2.
The signals in these relationships contain amplitude, frequency, and phase components, so it is useful to represent them as vectors. In simpler terms, it is also useful to represent these signals as vectors in a measurement system.
Measurement Challenges
The improvement in data throughput provided by MIMO technology has increased system complexity, bringing new design challenges to the test and measurement equipment used to evaluate MIMO systems and the components in the system. Before deciding on the best MIMO measurement instrument, it may be necessary to first determine the type of measurement that describes the performance of the MIMO communication channel. MIMO measurements can generally be divided into system-level measurements, channel response measurements, and functional measurements of the components used in the MIMO system.
It has been explained that MIMO signals are defined by frequency, amplitude and corresponding phase components. The measurement of MIMO signals must accurately and truly measure the above three signal characteristic components. In addition, MIMO systems are usually based on zero-IF down-conversion of received signals to baseband I and Q signal components. To obtain high modulation accuracy, the fidelity of I and Q signal components must be maintained, which requires all components in the signal path to have high performance and low distortion, including amplifiers, filters, mixers, I/Q modulators and demodulators.
In many wireless systems, error vector magnitude (EVM) is a standard parameter for evaluating performance and is widely used in MIMO systems. EVM, often referred to as the received signal constellation error (RCE), is intuitively displayed in the constellation diagram. RCE is actually the vector difference between the ideal signal and the measured signal, and can be used as a direct measure of the modulation accuracy and signal quality of the MIMO transmitter and receiver performance. The EVM measurement captures the signal amplitude and phase errors and reduces the many parameters that define the transmitted RF signal distortion to a single parameter, allowing comparisons between individual transmitters. Other important MIMO transmitter tests include the evaluation of group delay and group delay variation, phase noise, amplification compression, and I/Q mismatch of components in signal processing. Signal distortion caused by these factors can generally be seen through the EVM on the constellation diagram.
In the constellation diagram EVM, for an ideal signal, all constellation points should coincide exactly with the ideal position. However, signals and components are not perfect, and factors such as phase noise and carrier leakage can cause the constellation points on the constellation diagram to shift from the ideal position. EVM is a measurement of this shift. In addition to the overall EVM as a MIMO system test parameter, EVM as a frequency and EVM as a time function can also provide analysis of MIMO transmitter performance. In addition, the comparison of carriers and symbols displayed by EVM can provide further details on the performance of the MIMO transmitter.
The precise positioning of the points on the constellation EVM shows the performance of an excellent MIMO system. In a 2×2 MIMO system using OFDM and 64QAM, colors are used to distinguish between different transmitter signals and pilot carriers. In the constellation diagram shown in Figure 3, the red and blue dots represent the two signals in the 2×2 MIMO system, Tx0 and Tx1, which are overlaid on the white dots, which represent the ideal position of the subcarriers. The yellow dots represent the pilot carriers and overlap with the white dots representing the ideal pilot carriers.
Figure 3. The EVM constellation diagram provides a schematic representation of potential MIMO system problems, including noise (fuzzy dots), I/O imbalance (offset dots), and phase noise (dots that turn into rings).
Such color-defined diagrams make it easy to locate transmit signal problems. For example, red or blue subcarrier constellation points that are offset from the ideal white points indicate I/Q imbalance, while fuzzy constellation points indicate a noisy transmitted signal, and donut-shaped constellation points mean excessive phase noise.
Along with the more common XY plots, a range of channel measurements show the health of the plotting matrix and signal matrix relative to the subcarriers in the MIMO system. The measurement of the system's ability to flip the channel and transmit symbols in Figure 4 can be used to determine the orthogonality of the various signal streams in the MIMO system. By transmitting inverted symbols, the coverage of the system can be analyzed, and by transmitting parallel symbols, the system throughput can be evaluated.
Figure 4 XY diagram showing the orthogonality of MIMO channel subcarriers, with subcarriers marked [page]
The channel response measurement shows the flatness of the subcarriers, which are the subcarriers. For example, in a measurement on an IEEE 802.16e OFDM channel (shown in Figure 5), the green trace shows the power of the signal from the first transmitter (Tx0) to the first receiver (Rx0); the upper red trace shows the power of the signal from the second transmitter (Tx1) to the second receiver (Rx1); the blue trace shows the power of the signal from the first transmitter (Tx0) to the second receiver (Rx1); and the lower red trace shows the power of the signal from the second transmitter (Tx1) to the first receiver (Rx0). The power level of the corresponding subcarrier indicates the channel flatness, and the difference between the main signal and the indirect signal shows the channel isolation (less than 40dB in the example). These measurements are made by directly connecting the transmitter and receiver with a coaxial cable.
Figure 5: By directly connecting the MIMO transmitter and receiver, channel flatness and channel isolation can be evaluated. This example shows a 2×2 MIMO system.
A range of measurements in the time and frequency domains can show how MIMO performance changes under different circumstances. For example, EVM measurements against the OFDM symbol time can indicate interference issues or performance variations over time. EVM measurements against subcarriers can be used to analyze in-band noise effects, such as aliasing. Power measurements against the OFDM symbol time can isolate in-band amplitude deviations. Frequency measurements against the OFDM symbol time can be used to check frequency accuracy and isolate frequency drift issues over time within a packet.
Hardware construction
The test system for MIMO measurements must accurately simulate the operation of the MIMO system, generate the required signal frequency, amplitude and phase, and capture and analyze the signal in the test device (DUT). The test system must support the modulation format used and support all modulation bandwidths in the test. For the test signal generation process, an arbitrary waveform generator or vector signal generator (VSG) needs to provide control over the generation of actual test signals, and a vector signal analyzer (VSA) can be used as a test receiver. Any test system designed for MIMO systems should be able to provide the number of test signal sources and signal analyzers required for the number of paired transmitters and receivers, and should also be able to meet future upgrade requirements. For example, the MIMO test system provided by Keithley can be upgraded from a single VSG and VSA to an 8×8 channel system, and can flexibly configure the signal source and analyzer within that range.
If synchronization of multiple signal sources and analyzers is essential for MIMO measurements, then these instruments also require a common reference oscilloscope. For example, in the 2×2 MIMO measurement system from Keithley ( www.keithley.com ) shown in Figure 6, the VSA and VSG devices require specialized synchronization components. These components provide some common signals, such as local oscillators, common clocks, and precision triggers, and provide low sampling and RF carrier phase jitter, which is essential for accurate and repeatable measurements of OFDM MIMO signals. In particular, the synchronization components provide less than 1° peak-to-peak jitter.
Figure 6. This MIMO test system is based on a multi-channel vector signal generator (VSG), a vector signal analyzer (VSA), and synchronization components controlled by a computer and custom measurement software.
The effectiveness and ease of use of a MIMO test system also depends on the system's test software. As MIMO technology continues to be adopted in wireless communication systems, off-the-shelf test software is becoming more common in simplifying system and channel measurements. For example, Keithley's SignalMeisterRF Communications Test Toolkit Model 290101 provides complex signal generation and signal analysis capabilities for MIMO applications such as WLAN 802.11n and WIMAX 802.16e Wave 2. This software package works seamlessly with Keithley's VSG, VSA, and MIMO synchronization components to form a complete measurement system for complex communication systems. In addition to EVM and MIMO channel response measurements, the software can also handle the evaluation of SISO systems.
The tests and measurements we have discussed so far can be used to evaluate the performance of MIMO communication systems and components in the system under ideal conditions. But how does the MIMO system perform under weak signal conditions? In this case, a different type of test is needed, such as a channel simulator. It provides methods for analyzing MIMO systems and components under channel impairments, such as signal attenuation, Gaussian white noise (AWGN), channel crosstalk, and even Doppler effects - usually caused by the movement of the communication terminal in the car relative to the base station.
The channel simulator must function as both a transmitter and receiver in a MIMO system and must have the ability to simulate real-world conditions such as signal attenuation and delay. A qualified channel simulator also provides software-defined radio modules, such as ITU M.1225 A and B in WiMAX. A practical channel simulator must exceed the performance of the system under test and provide the ability to be used for production testing when needed. The simulator also needs to have bidirectional capabilities so that it can provide both uplink and downlink testing. By also providing calibrated reciprocal tests, the simulator is very useful for testing MIMO systems that use beamforming techniques. Finally, although the examples shown in this article are for 2×2 MIMO systems, an effective channel simulator can also support 4×4 MIMO systems to achieve complete support for a variety of MIMO systems. For example, the ACE 400WB channel simulator from Azimuth Systems ( www.azimuthsystems.com ) is a bidirectional component that supports testing of 4×4 MIMO systems.
Previous article:Application of MAX262 filter in underground metal pipeline detector
Next article:A crosstalk fault detection scheme in GSM base stations
Recommended ReadingLatest update time:2024-11-16 20:22
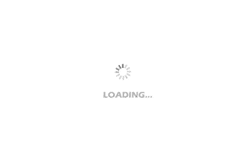
- Popular Resources
- Popular amplifiers
-
Real-time driver monitoring system via modal and viewpoint analysis
-
Evaluating Roadside Perception for Autonomous Vehicles: Insights from Field Testing
-
Convex Optimization in Signal Processing and Communications: From Foundations to Applications (Qi Zhongyong, Li Weichang, Lin Jiaxiang)
-
A Practical Tutorial on ASIC Design (Compiled by Yu Xiqing)
- Keysight Technologies Helps Samsung Electronics Successfully Validate FiRa® 2.0 Safe Distance Measurement Test Case
- From probes to power supplies, Tektronix is leading the way in comprehensive innovation in power electronics testing
- Seizing the Opportunities in the Chinese Application Market: NI's Challenges and Answers
- Tektronix Launches Breakthrough Power Measurement Tools to Accelerate Innovation as Global Electrification Accelerates
- Not all oscilloscopes are created equal: Why ADCs and low noise floor matter
- Enable TekHSI high-speed interface function to accelerate the remote transmission of waveform data
- How to measure the quality of soft start thyristor
- How to use a multimeter to judge whether a soft starter is good or bad
- What are the advantages and disadvantages of non-contact temperature sensors?
- Innolux's intelligent steer-by-wire solution makes cars smarter and safer
- 8051 MCU - Parity Check
- How to efficiently balance the sensitivity of tactile sensing interfaces
- What should I do if the servo motor shakes? What causes the servo motor to shake quickly?
- 【Brushless Motor】Analysis of three-phase BLDC motor and sharing of two popular development boards
- Midea Industrial Technology's subsidiaries Clou Electronics and Hekang New Energy jointly appeared at the Munich Battery Energy Storage Exhibition and Solar Energy Exhibition
- Guoxin Sichen | Application of ferroelectric memory PB85RS2MC in power battery management, with a capacity of 2M
- Analysis of common faults of frequency converter
- In a head-on competition with Qualcomm, what kind of cockpit products has Intel come up with?
- Dalian Rongke's all-vanadium liquid flow battery energy storage equipment industrialization project has entered the sprint stage before production
- Allegro MicroSystems Introduces Advanced Magnetic and Inductive Position Sensing Solutions at Electronica 2024
- Car key in the left hand, liveness detection radar in the right hand, UWB is imperative for cars!
- After a decade of rapid development, domestic CIS has entered the market
- Aegis Dagger Battery + Thor EM-i Super Hybrid, Geely New Energy has thrown out two "king bombs"
- A brief discussion on functional safety - fault, error, and failure
- In the smart car 2.0 cycle, these core industry chains are facing major opportunities!
- The United States and Japan are developing new batteries. CATL faces challenges? How should China's new energy battery industry respond?
- Murata launches high-precision 6-axis inertial sensor for automobiles
- Ford patents pre-charge alarm to help save costs and respond to emergencies
- New real-time microcontroller system from Texas Instruments enables smarter processing in automotive and industrial applications
- Problems with synchronous operation of two or more DC brushed motors with dual Hall
- How to use the address filtering function in the Tri-Speed Ethernet IP core
- TI Wireless Products RF Hardware FAQ - 13 new webinar Q/A summaries
- Quectel BC28 NB module cannot read SIM card
- Why does CCS make a thumping sound and then disconnect when it is running?
- TI: mmWave sensors enable intelligence at the edge
- Where can I buy the EP3C25Q240C8N development board? How much is it worth?
- Programming Example: DSP Realizes SVPWM Waveform
- FPGA Advanced Timing Synthesis Tutorial
- TI will work with you to create a new driving experience in the future - experience it yourself: The limited-time purchase event for star products has begun