LiDAR is rapidly gaining attention and deployment in ADAS and autonomous vehicle sensing systems, but there are different approaches to implementing the technology. This article explains these approaches and the relative advantages of coherent LiDAR detection.
Light detection and ranging (lidar) was first conceptualized in the 1930s, around the same time as radio detection and ranging (radar). However, it was not until the advent of lasers in the 1960s that the technology was demonstrated. In the years that followed, the development of optical communications led to major advances in laser and light modulation technology.
The first commercial LiDAR system, originally known as “Lidar,” debuted in Volvo passenger cars in 2008. This groundbreaking technology powered one of the first Autonomous Emergency Braking (AEB) systems, allowing vehicles to automatically brake to prevent or mitigate rear-end collisions.
Following its early introduction 15 years ago (and subsequently replaced by radar as a cheaper alternative to AEB), high-resolution lidar has rapidly evolved into a key high-resolution sensor for autonomous vehicle programs and has spawned several innovative, well-funded startups. Offering extended range, superior resolution, and real-time 3D visualization of the vehicle's surroundings, the technology has now matured into an important sensor modality not only for autonomous driving, but also as a complement to advanced driver assistance systems (ADAS) for passenger cars and commercial fleets.
LiDAR sensors emit photons in the infrared spectrum to detect and create a 3D image of the surrounding environment. They have proven to be very popular in automotive applications. The main advantage of LiDAR compared to radar is the very short wavelength of light used, which allows for precise measurements. In addition, LiDAR can work in any lighting conditions and has a better detection range compared to cameras. LiDAR sensor data capture can be viewed as a “point cloud” (see Figure 1).
There are many things to consider when developing a LiDAR system, such as what wavelength to use, the scanning method, and how to deal with interference. However, the biggest system decision is how to best detect the returning photons. There are two main contenders, direct detection vs. coherent detection.
Direct detection
In a direct detection system, transmitting a laser pulse effectively starts a timer. When the echo of the laser pulse is received, it stops and the distance is calculated based on the elapsed time. See Figure 2.
Figure 2: Since the speed of light (c) is constant, the distance to the target is δTC/2, where t is the time between the start of photon transmission and the leading edge of photon reception.
For distances up to about 50 m, there is no need for a high-quality tunable single-mode laser (since it is simply a source of a large number of photons compressed into a short time) or modulation, which simplifies the driver circuitry. There is also no need for precision optics to compensate for wavefront distortion.
Why short range? As the illuminated area increases with distance, the returned power decreases (distance squared). Note: The formula is: returned power is approximately equal to transmitted power x (target area / illuminated area) x (receiving area / (π x range2)). This loss cannot be avoided, so the simplest solution is to transmit more power or increase receiver sensitivity.
However, the laser energy that can be used is limited. Intense near-infrared (IR) light (800 to 1400 ηm) can damage human vision. In ADAS or self-driving car applications, simply increasing the transmitted power of near-IR light can create dangers for other road users and pedestrians.
As for improving receiver sensitivity, a larger area receiving lens can be used to increase photon collection. In addition, avalanche photodiodes (APDs, photodiodes with inherent gain) can be used, although they tend to be expensive, fragile, and small (which further complicates the system optics) and only provide about 15 times gain before self-generated noise becomes a problem. Other sensor types, such as Geiger-mode avalanche photodetectors (GMAPDs) and single-photon avalanche detectors (SPADs) provide better sensitivity in direct detection lidar systems, but do not work well in snowy, dusty, or foggy environments.
Additionally, all detection systems require some form of anti-interference measures. Whether it’s radar or lidar, the systems need to know that the signals their receivers are picking up (whether pulsed radio waves or photons) came from their transmitters. Interference issues arose in early pulsed automotive radars. Once many cars were equipped with radars, mutual interference became a problem. The most popular solution was to switch to coherent detection technology, primarily frequency modulated continuous wave (FMCW – see below) for radar systems.
Another limitation of direct detection lidar is that it cannot directly measure velocity at every point, but must calculate velocity by determining how distance changes over time (i.e., comparing multiple subsequent frames), which can affect the responsiveness of the system.
Coherent Detection and FMCW
This involves mixing the incident light with a sample of the transmitted light, which has two main benefits. First, noise-free amplification through photon gain can be achieved via constructive interference (i.e., the received signal is multiplied by the transmitted signal), allowing the system to achieve excellent sensitivity with very low-power lasers. Second, mixing the transmitted and received signals makes the lidar system very selective, as light that is not exactly the same wavelength (such as sunlight or light from neighboring lidar systems) is simply ignored.
There are different ways to implement a coherent detection lidar system, but the most common is frequency modulated continuous wave (FMCW) modulation. Figure 3 shows a simplified example.
Figure 3: The laser operates at around 1550ηm and is modulated by a few hundred MHz (e.g., from 1550.002 to 1550ηm). The transmitted signal (and reflected signal) is around 200 Hz. After light mixing, the photodiode presents the sum and difference of the two signals. The photodiode has limited bandwidth and is non-responsive to around 400THz and can only detect differential signals of a few hundred MHz.
In effect, the laser sweeps up and down in frequency, producing a sawtooth profile (frequency versus time) from which both distance and velocity can be derived; for the latter, think of the Doppler effect. Figure 4 shows a more detailed overview of the optical components.
Figure 4: The main optical components of an FMCW lidar system.
Although more complex than direct detection systems, the benefits of FMCW lidar are numerous. For example, as mentioned above, the return signal is multiplied by the samples taken from the transmitting source (local oscillator, LO in Figure 4). Due to the high path loss of lidar, even a few percent of the LO will be much larger than the return signal. The signal amplification is very high, but only for signals of exactly the same wavelength, which leads to high photon efficiency.
For example, an FMCW lidar system with a range of about 300 meters can be achieved with less than 200 mW of laser power. A similar direct detection system would require 1000 times more peak power for the same range. It should be noted that FMCW is at the heart of lidar in other sectors; for example, optical altimeter instruments have ranges of up to several thousand meters and laser Doppler lidar instruments have ranges of over 500 meters.
Another benefit of coherent lidar is that the bandwidth of the signal chain is quite low. If we consider the wavelength in Figure 3 (the laser sweep range is 1550.002 to 1550ηm), the photodiode bandwidth can be limited to a few hundred MHz. In order to resolve the leading edge of the received pulse, the direct detection system needs as wide a bandwidth as possible (usually more than 2GHz).
As can be appreciated, a narrower bandwidth allows the use of a lower noise transimpedance amplifier and a slower analog-to-digital converter at the photodiode.
Finally, coherent detection provides velocity information at each point. The benefit of velocity at each point is that it is an additional contextual metric that subsequent perception systems can use when interpreting lidar (and other sensor) data, potentially enabling more intelligent decisions.
The various benefits of coherent detection are therefore significant, but coherent lidar is not without its challenges.
The laser must be able to maintain its phase integrity long enough for its light to reach the most distant target and return from that target. If the phase of the laser varies greatly during the transmission time, coherence may be lost and the distance measurement may be ambiguous. In addition, it must be frequency modulated (in the case of FMCW). Most diode lasers are not up to this task, but some semiconductor tunable lasers have appeared on the commercial market.
Furthermore, not every scanning mechanism is compatible with coherent detection. The receiver needs to observe each light spot long enough to allow the light to reach and return from the target as far away as possible, because the return signal needs to be mixed with the transmitted signal. For example, a range of 300 meters requires the scanning mechanism to remain stationary for at least 2 seconds, but many scanning mechanisms that move continuously cannot do this.
Finally, it is important to note that the signal processing tasks for coherent lidar are significantly greater than for direct detection. Fortunately, semiconductor manufacturers have introduced high-performance system-on-chip (SoC) products that integrate data converters, microcontrollers, and DSPs with FFT accelerators to meet these signal processing needs: indie Semiconductor's iND83301 Surya lidar SoC is one such example.
Previous article:Comparison of Multimodal Data Perception Fusion Solutions for Autonomous Driving
Next article:What are the advantages of bidirectional charging for electric vehicles?
Recommended ReadingLatest update time:2024-11-16 14:49
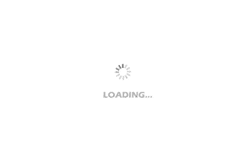
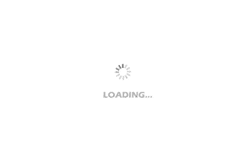
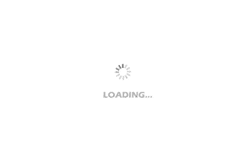
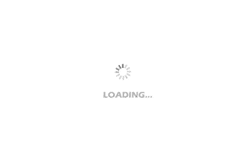
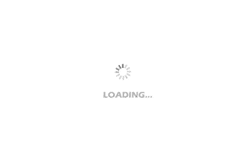
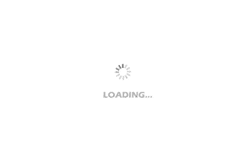
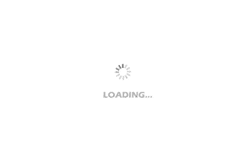
- Popular Resources
- Popular amplifiers
-
LiDAR point cloud tracking method based on 3D sparse convolutional structure and spatial...
-
Distributed robust Kalman filter fusion algorithm for ADAS system vision and millimeter wave radar
-
GenMM - Geometrically and temporally consistent multimodal data generation from video and LiDAR
-
Comparative Study on 3D Object Detection Frameworks Based on LiDAR Data and Sensor Fusion Technology
- Huawei's Strategic Department Director Gai Gang: The cumulative installed base of open source Euler operating system exceeds 10 million sets
- Analysis of the application of several common contact parts in high-voltage connectors of new energy vehicles
- Wiring harness durability test and contact voltage drop test method
- Sn-doped CuO nanostructure-based ethanol gas sensor for real-time drunk driving detection in vehicles
- Design considerations for automotive battery wiring harness
- Do you know all the various motors commonly used in automotive electronics?
- What are the functions of the Internet of Vehicles? What are the uses and benefits of the Internet of Vehicles?
- Power Inverter - A critical safety system for electric vehicles
- Analysis of the information security mechanism of AUTOSAR, the automotive embedded software framework
Professor at Beihang University, dedicated to promoting microcontrollers and embedded systems for over 20 years.
- Innolux's intelligent steer-by-wire solution makes cars smarter and safer
- 8051 MCU - Parity Check
- How to efficiently balance the sensitivity of tactile sensing interfaces
- What should I do if the servo motor shakes? What causes the servo motor to shake quickly?
- 【Brushless Motor】Analysis of three-phase BLDC motor and sharing of two popular development boards
- Midea Industrial Technology's subsidiaries Clou Electronics and Hekang New Energy jointly appeared at the Munich Battery Energy Storage Exhibition and Solar Energy Exhibition
- Guoxin Sichen | Application of ferroelectric memory PB85RS2MC in power battery management, with a capacity of 2M
- Analysis of common faults of frequency converter
- In a head-on competition with Qualcomm, what kind of cockpit products has Intel come up with?
- Dalian Rongke's all-vanadium liquid flow battery energy storage equipment industrialization project has entered the sprint stage before production
- Allegro MicroSystems Introduces Advanced Magnetic and Inductive Position Sensing Solutions at Electronica 2024
- Car key in the left hand, liveness detection radar in the right hand, UWB is imperative for cars!
- After a decade of rapid development, domestic CIS has entered the market
- Aegis Dagger Battery + Thor EM-i Super Hybrid, Geely New Energy has thrown out two "king bombs"
- A brief discussion on functional safety - fault, error, and failure
- In the smart car 2.0 cycle, these core industry chains are facing major opportunities!
- The United States and Japan are developing new batteries. CATL faces challenges? How should China's new energy battery industry respond?
- Murata launches high-precision 6-axis inertial sensor for automobiles
- Ford patents pre-charge alarm to help save costs and respond to emergencies
- New real-time microcontroller system from Texas Instruments enables smarter processing in automotive and industrial applications
- What is the use of this circuit? The input of the previous stage is the level of the comparator output
- Help with AD18 pin display problem!!
- How to choose the right Cortex-based MCU application design
- C8051F340 Development Board
- "【TGF4042 Signal Generator】" Circuit Parameter Measurement
- EEWORLD University - Texas Instruments Deep Learning (TIDL) Overview
- Can the transmission speed of Zigbee be achieved on a small car?
- Applying Over-the-Air Firmware Upgrades to MSP 430 Microcontrollers
- LED glass screen
- Download and get a gift! Meet spring and have fun with us! Come and learn about optical communication test and measurement solutions with Keysight