By: Diarmúid Carey, Applications Engineer, Analog Devices
question:
Are there any active circuit protection solutions that can replace TVS diodes and fuses?
Answer:
You could try a surge suppressor.
summary
Manufacturers in all industries are constantly pushing for higher-end performance while trying to balance such innovation with proven, reliable solutions. Designers face the difficult task of balancing design complexity, reliability, and cost. Take an electronic protection subsystem, for example, which is limited by its nature and cannot afford innovation. These systems protect sensitive and expensive downstream electronics (FPGAs, ASICs, and microprocessors), which all require zero-failure guarantees.
Many traditional reliable protection solutions (such as diodes, fuses and TVS devices) can maintain the protection state, but they are usually inefficient, bulky and require maintenance. To address these shortcomings, active smart protection ICs have emerged, which can meet the protection requirements of traditional methods and are more reliable in some aspects. However, there are many types of devices, so the most difficult problem facing designers is to choose the right solution.
To help designers narrow their choices, this article compares traditional protection methods and the ADI protection product portfolio to showcase the features of these products and suggested applications.
Introduction
As the number of electronic devices used in all industries continues to increase, and the processing capabilities of expensive FPGAs and processors continue to expand, there is an increasing demand for protection of these devices operating in harsh environments. In addition, they need to be small, reliable, and able to respond quickly to overvoltage and overcurrent surge events. This article explores the challenges faced by many applications and why protection is needed, comparing traditional protection methods with newer alternative solutions that provide greater accuracy, reliability, and design flexibility.
Why consider using voltage and current protection devices?
Automotive, industrial, communications, and avionics systems are subject to a range of power surges, such as those shown in Figure 1. In these markets, transient events are defined in a number of industry specifications. For example, the ISO 7637-2 and ISO 16750-2 specifications define automotive transients, outlining in detail the transients to expect, as well as the test procedures to ensure they are consistently validated.
The type and energy of surge events will vary depending on the area in which the electronics are used; circuits may be subject to overvoltage, overcurrent, reverse voltage, and reverse current. Ultimately, many circuits would not be able to sustain, let alone operate independently, if directly subjected to these transient conditions shown in Figure 1, so designers must consider all input conditions and implement mechanisms that can protect circuits from voltage and current surges.
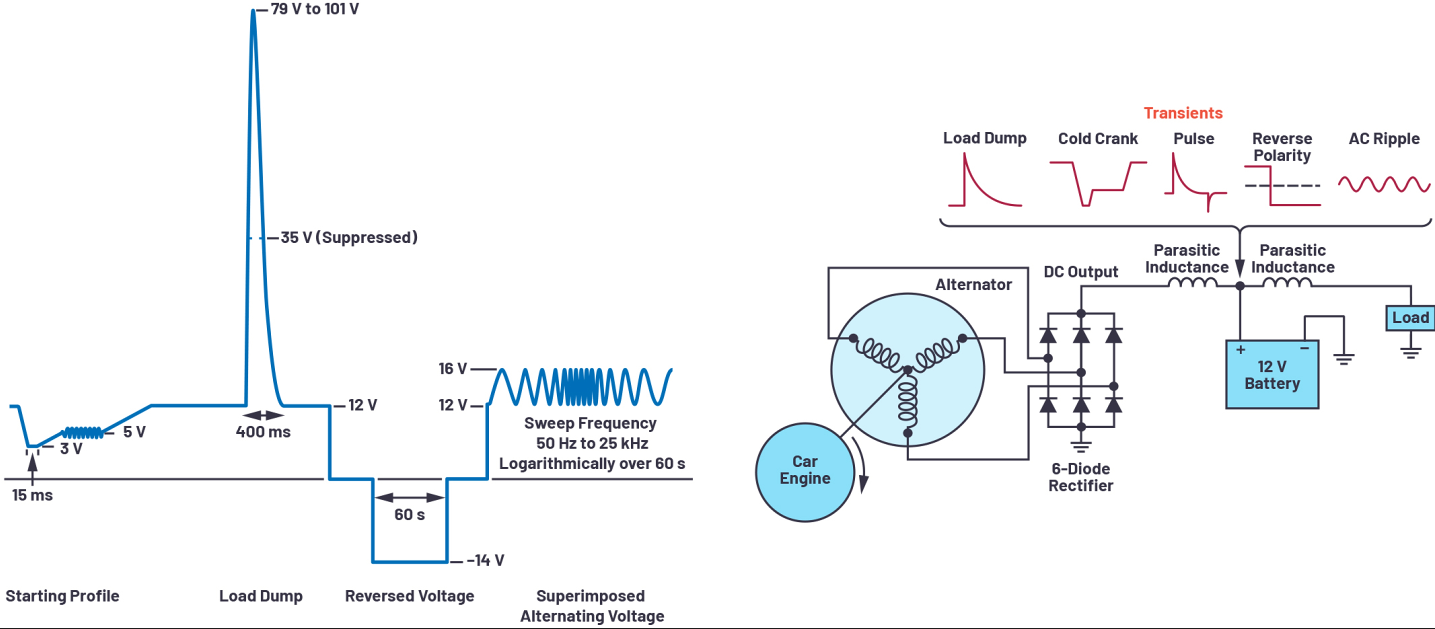
Figure 1. Overview of some of the more stringent ISO 16750-2 tests
Design Challenges
There are many different causes for transient voltages and currents in electronic systems, but some electronic environments are more prone to transient events than others. It is well known that applications in automotive, industrial, and communications environments are subject to potentially harmful events that can cause severe damage to downstream electronics, but surge events are not limited to these environments. Other situations that may require surge protection circuits include applications that require high voltage or high current power supplies, applications that use hot-swappable power connections, or systems that contain motors or may be subject to lightning-induced transients. High voltage events can last anywhere from microseconds to hundreds of milliseconds, so flexible and reliable protection mechanisms must be in place to ensure the longevity of costly downstream electronics.
For example, an automotive load dump occurs when the alternator (which charges the battery) is temporarily disconnected from the battery. When this disconnection occurs, the full charging current from the alternator is delivered to the power rail, causing the rail voltage to climb to very high levels (>100 V) within hundreds of milliseconds.
There are many reasons why surges can occur in communications applications, ranging from hot-swapping communications cards to outdoor installations that may be affected by lightning. Long cables used in large facilities can also cause induced voltage spikes.
Ultimately, designers must fully understand the environment in which the device will be used and meet existing regulatory requirements. This helps them take into account all fault types to adopt the best protection mechanism, making it reliable and non-intrusive, but allowing downstream electronics to operate within safe voltage ranges with minimal disruption.
Traditional protection circuit
With so many different types of electronic issues to consider, how should electronics engineers protect sensitive downstream electronics?
Traditional protection methods are based on multiple devices to provide protection instead of one, for example, using transient voltage suppressors (TVS) for overvoltage protection, line fuses for overcurrent protection, series diodes for reverse battery/power protection, and a mix of capacitors and inductors to filter lower energy spikes. Although discrete configurations can meet established specifications (protecting downstream circuits), it is cumbersome to implement and requires multiple selections to determine the appropriate filtering specifications.
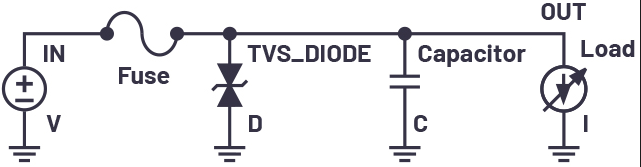
Figure 2. Traditional protection devices
Let’s take a closer look at these devices to understand the advantages and disadvantages of this implementation approach.
TVS - Transient Voltage Suppressor
This is a relatively simple device that protects downstream circuitry from high voltage spikes on a power supply. They can be classified into several different types with a wide range of characteristics (Table 1 lists them in order from shortest to longest response time).
Table 1. Response time of different transient voltage suppression devices

Although their structures and characteristics vary, their usage is similar: when the voltage exceeds the device threshold, the excess current is diverted. TVS can fix the output voltage at the rated level in a very short time. For example, the response time of TVS diodes can be as low as picoseconds, while the response time of GDTs may be several microseconds, but they can handle larger surges.
Figure 3 shows a simple configuration of a TVS diode for protecting downstream circuitry. Under normal operating conditions, the TVS has high impedance and the input voltage is passed directly to the output. When an overvoltage appears at the input, the TVS begins to conduct and shunts the excess energy to ground (GND), thereby clamping the downstream load voltage. The power rail voltage rises above the typical operating value, but is clamped to a value that ensures that the downstream circuitry can operate safely.
While TVS devices are effective at suppressing very high voltage excursions, they are not immune to damage when subjected to sustained overvoltages, and therefore require regular monitoring or replacement. Another concern is that the TVS could short out, disconnecting the input power supply. Additionally, depending on the amount of energy involved, they may need to be sized large to meet margin requirements, resulting in a corresponding increase in solution size. Even if the TVS is sized correctly, the downstream circuitry must be able to handle the clamping voltage, increasing the voltage rating requirements downstream.
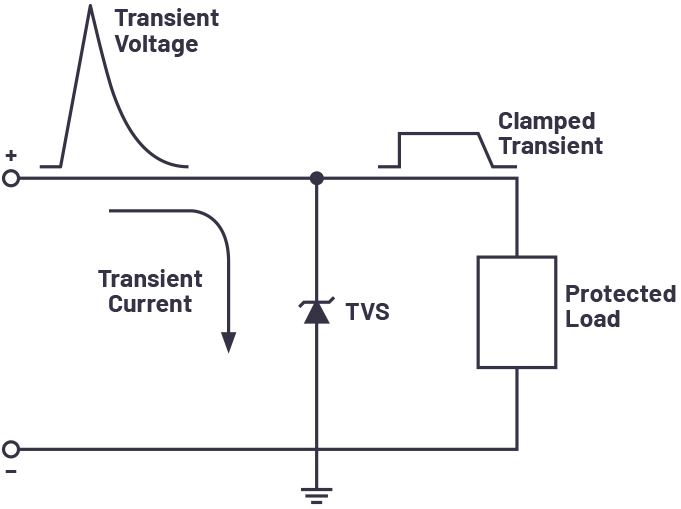
Figure 3. Protecting against voltage surges with a traditional TVS solution
Line fuse
Overcurrent protection can be implemented using common line fuses with a higher-than-nominal breaking rating, for example, 20% higher than the maximum rated current (the percentage depends on the type of circuit and the expected typical operating load). Of course, the biggest problem with fuses is that they must be replaced once they have blown. Fuses are fairly simple in design, but maintenance is relatively complex, especially in hard-to-reach locations, so it is still time-consuming and costly later. Maintenance requirements can be reduced by using backup fuses, such as resettable fuses, which use a positive temperature coefficient to open the circuit when a current higher than the nominal current flows through the device (the increase in current causes the temperature to increase, resulting in a sharp increase in resistance).
Aside from maintenance issues, one of the biggest issues with fuses is their reaction time, which can vary greatly depending on the type of fuse selected. We can use fast-acting fuses, but the fusing time (the time to open the circuit) can still take hundreds of microseconds to milliseconds, so circuit designers must consider the amount of energy released during these time periods to ensure that downstream electronics are not damaged.
Series diode
In some environments, a circuit may be disconnected and then reconnected—for example, in a battery-powered environment. In this case, there is no guarantee that the polarity is correct when the power is reconnected. Polarity protection can be achieved by adding a series diode to the positive supply line of the circuit. While this simple addition is effective in preventing reverse polarity, there is a corresponding power loss due to the voltage drop across the series diode. In relatively low current circuits, this trade-off is small, but for many modern, high current rails, another solution is needed. Figure 4 is an update to Figure 3 showing the use of a TVS and the addition of a series diode to protect against reverse polarity connection.
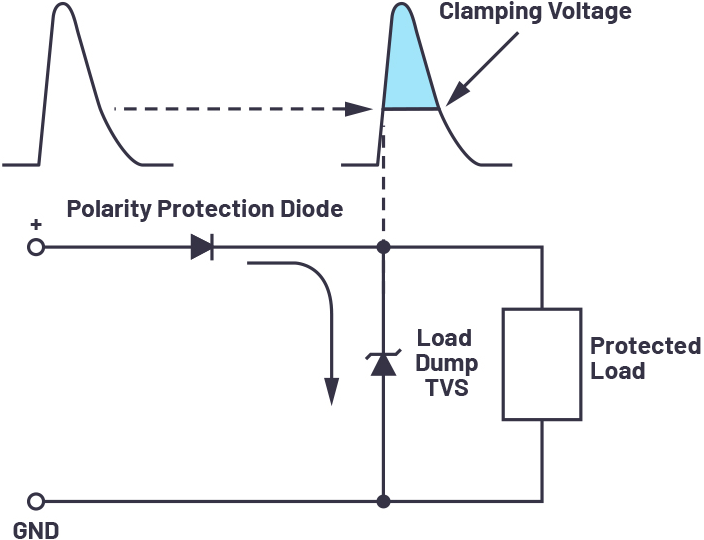
Figure 4. Adding a series diode can prevent reverse polarity connection, but in high current systems, the voltage drop across the diode can be a problem.
Filters using inductors and capacitors
The passive solutions discussed so far all work by limiting the amplitude, but they usually only capture larger amplitudes and miss smaller spikes. These smaller transients can still cause damage to downstream circuits, so additional passive filters are needed to smooth out the spikes. This can be achieved by using discrete inductors and capacitors, sizing them so that they attenuate voltages that are out of frequency. Before design, the filter designs need to be tested and measured to determine their size and frequency before the filter can be properly sized. The disadvantage of this approach is that it is necessary to consider material cost and area requirements (how much board area and cost of components must be achieved to achieve the filtering level), as well as whether over-design is required (determining the tolerances of components to provide compensation when they change over time and temperature).
Using Surge Suppressors for Active Protection
One way to overcome the challenges and drawbacks of the passive protection solutions described above is to switch to surge stopper ICs. Surge stoppers use easy-to-use controller ICs and series N-channel MOSFETs, eliminating the need for complex shunt circuits (TVS devices, fuses, inductors, and capacitors). Surge stopper controllers can greatly simplify system design because only a few components need to be sized and qualified.
The surge stopper continuously monitors the input voltage and current. Under nominal operating conditions, the controller drives the gate of the N-channel MOSFET pass device fully on, providing a low impedance path from input to output. In the event of an overvoltage or surge (the threshold is given by the feedback network at the output), the IC regulates the gate of the N-channel MOSFET, clamping the MOSFET's output voltage to the level set by the resistor divider.
Figure 5 shows a simplified schematic of a surge stopper configuration and the results when a 100 V input surge occurs on a nominal 12 V rail. During the surge, the output of the surge stopper circuit is clamped to 27 V. Some surge stoppers also use a series sense resistor (circuit breaker in Figure 5) to monitor for overcurrent conditions and adjust the gate of the N-channel MOSFET to limit the current at the output load.
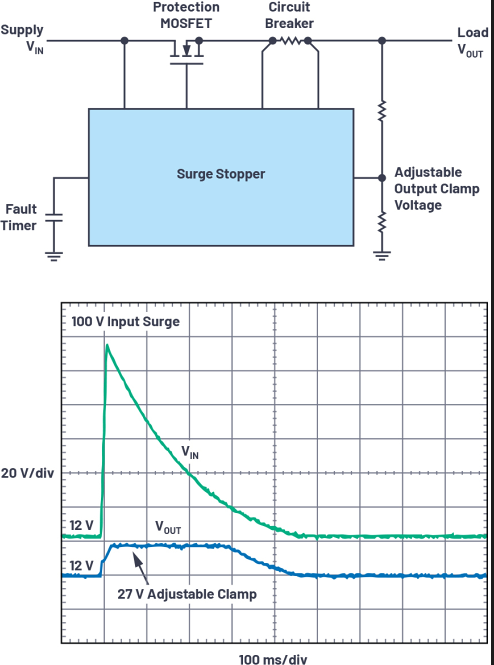
Figure 5. Detailed schematic of surge suppressor configuration
Surge suppressors can be divided into four main categories based on their response to overvoltage events:
Linear Surge Suppressor
Gate clamping
Switching Surge Suppressor
Output disconnect protection controller
Surge suppressors should be selected based on the application, so let's compare their operation and advantages.
Surge suppressor type: Linear
The linear surge stopper drives the series MOSFET in a similar way to a linear regulator, limiting the output voltage to a preset safe value and dissipating excess energy in the MOSFET. To protect the MOSFET, the device uses a capacitor fault timer to limit the time spent in the high dissipation region.
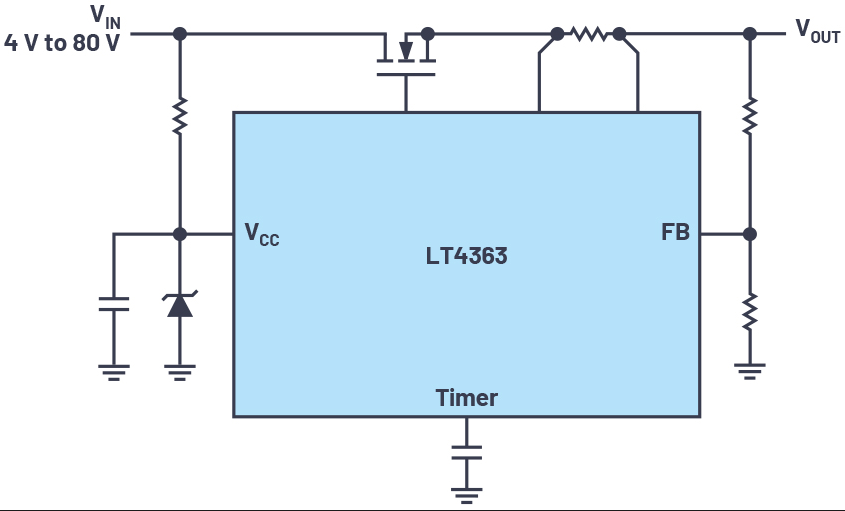
Figure 6. LT4363 linear surge stopper
Surge Stopper Type: Gate Clamp
A gate clamp surge stopper limits the voltage at the gate pin to this voltage using an internal or external clamp (e.g., 31.5 V or 50 V internal clamp, or an adjustable external clamp), and the output voltage limit is then determined by the threshold voltage of the MOSFET. For example, when using an internal 31.5 V gate clamp and the MOSFET threshold voltage is 5 V, the output voltage is limited to 26.5 V. Alternatively, an external gate clamp allows for a wider range of voltage choices. An example of a gate clamp surge stopper is shown in Figure 7.
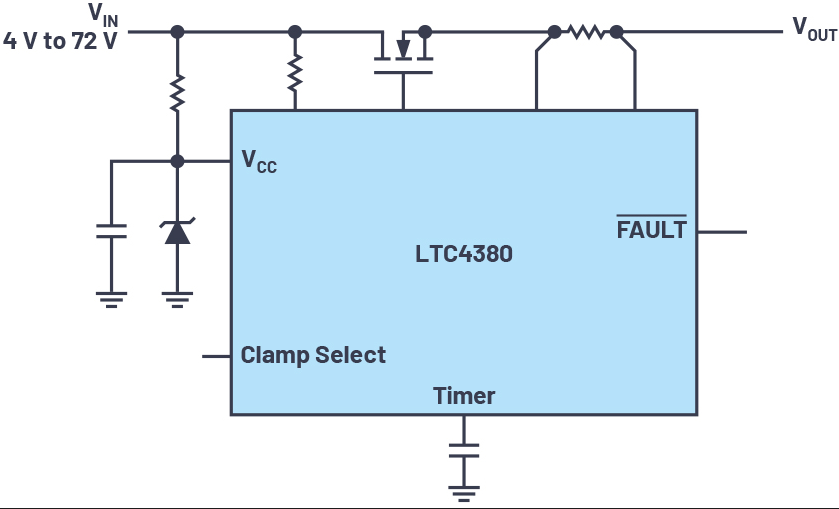
Figure 7. LTC4380 gate-clamped surge stopper.
Surge Suppressor Type: Switching
For higher power applications, a switching surge stopper is a good choice. Like linear and gate clamp surge stoppers, a switching surge stopper fully enhances the pass FET under normal operating conditions to provide a low resistance path between the input and output (minimizing power loss). The main difference between a switching surge stopper and a linear or gate clamp surge stopper occurs when a surge event is detected. During a surge event, a switching surge stopper regulates the output to the clamp voltage by switching the external MOSFET (much like a switching DC-DC converter).
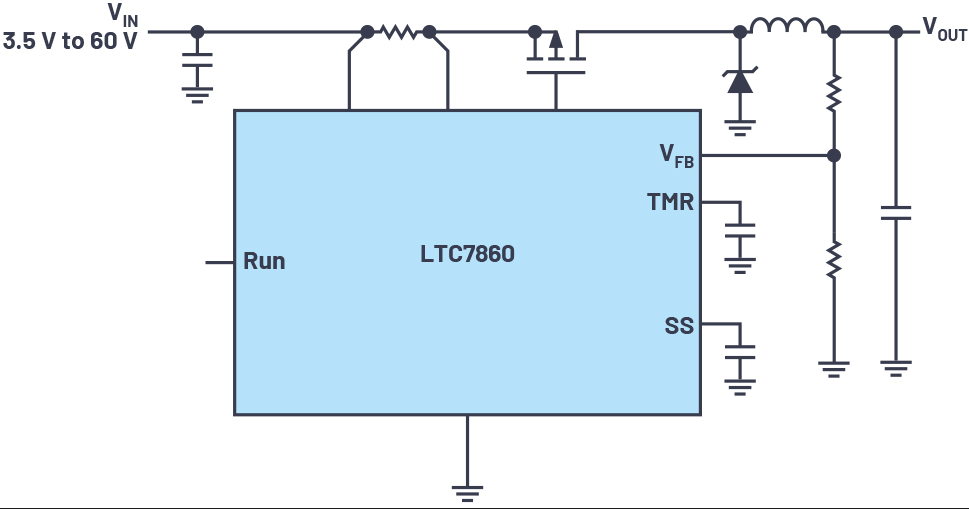
Figure 8. LTC7860 switching surge suppressor
Protection controller: Output disconnect
A protection controller is not a true surge stopper, but it does stop a surge. Like a surge stopper, a protection controller monitors for overvoltage and overcurrent conditions, but it does not clamp or regulate the output. Instead, it protects downstream electronics by immediately disconnecting the output. This simple protection circuit has a compact layout and is ideal for portable battery-powered applications. A simplified schematic of the LTC4368 protection controller and its response to an overvoltage event is shown in Figure 9. There are many versions of protection controllers.
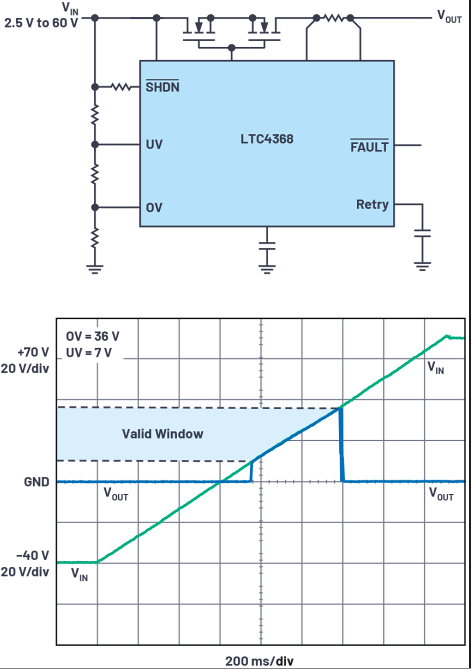
Figure 9. LTC4368 protection controller
The protection controller monitors the input voltage to ensure that the voltage remains within the voltage range configured by the resistor divider at the OV/UV pin, and disconnects the output using back-to-back MOSFETs when the input voltage exceeds this range, as shown in Figure 9. Back-to-back MOSFETs can also be used to protect against reverse input. The sense resistor at the output implements overcurrent protection by continuously monitoring the forward current, but does not require a timer-based ride-through operation.
Surge Suppressor Characteristics
To choose the best surge stopper for your application, you need to know what features are available and what challenges they can help solve. You can find these devices in parameter tables.
Disconnection and crossing
Some applications require disconnecting the output from the input when a surge event is detected. In this case, disconnecting the overvoltage connection is required. If you need the output to remain operational during a surge event to minimize downtime for downstream electronics, a surge stopper is required to ride through the surge. In this case, using a linear or switching surge stopper can accomplish this function (assuming the power level is reasonable for the topology and FETs selected).
Fault Timer
When implementing ride-through, the MOSFET needs to be protected from sustained surges. To ensure staying within the safe operating area (SOA) of the FET, a timer can be used. The timer is essentially a capacitor to ground. When an overvoltage occurs, an internal current source begins charging this external capacitor. When the capacitor reaches a certain threshold voltage, the digital fault pin pulls low, indicating that the pass transistor will soon shut down due to the prolonged overvoltage. If the timer pin voltage continues to rise to the secondary threshold, the gate pin will pull low to turn off the MOSFET.
The rate of change of the timer voltage varies with the voltage across the MOSFET, that is, the higher the voltage, the shorter the time, and the lower the voltage, the longer the time. This useful feature allows the device to ride out short overvoltage events smoothly, allowing downstream components to continue to operate while protecting the MOSFET from damage caused by longer-lasting overvoltage events. Some devices have a retry function that allows the device to turn the output on again after cooling down.
Overcurrent protection
Many surge stoppers have the ability to monitor current and protect the device from overcurrent events. This is accomplished by monitoring the voltage drop across a series sense resistor and responding appropriately. MOSFETs can also be protected by monitoring and controlling inrush current. The response can be similar to an overvoltage condition in that if the circuit can accept the power level, it will either disconnect by latching off or by a ride-through event.
Reverse input protection
Surge stoppers have a wide operating capability (able to withstand voltages up to 60 V below ground on some devices), so reverse input protection is possible. Figure 10 shows a back-to-back MOSFET configuration that provides reverse current protection. During normal operation, Q2 and Q1 are turned on by the gate pins, and Q3 has no effect. However, in the presence of a reverse voltage connection, Q3 turns on, pulling the gate of Q2 down to the negative input and isolating Q1 to protect the output.
Reverse output voltage protection is also achieved through robust device pin protection, which can withstand ground voltages up to 20 V, depending on the device selected.
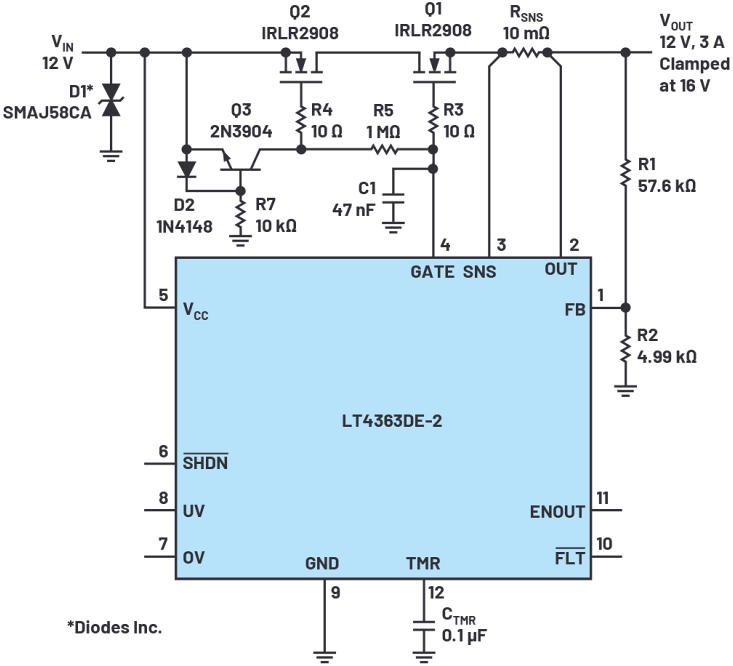
Figure 10. LT4363 reverse input protection circuit
For applications that require a wide input voltage range, a floating topology surge stopper can be used. When a surge event occurs, the surge stopper IC monitors the entire surge voltage, limiting the voltage range of the IC by internal transistor technology. When using a floating surge stopper (such as the LTC4366), the IC floats just below the output voltage, giving it a wider operating voltage range. A resistor (VSS) is included in the power return line to allow the IC to float with the supply voltage. In this way, the input voltage limit is set by the external components and the voltage function of the MOSFET. The application circuit shown in Figure 11 can operate normally with very high DC power while protecting downstream loads.
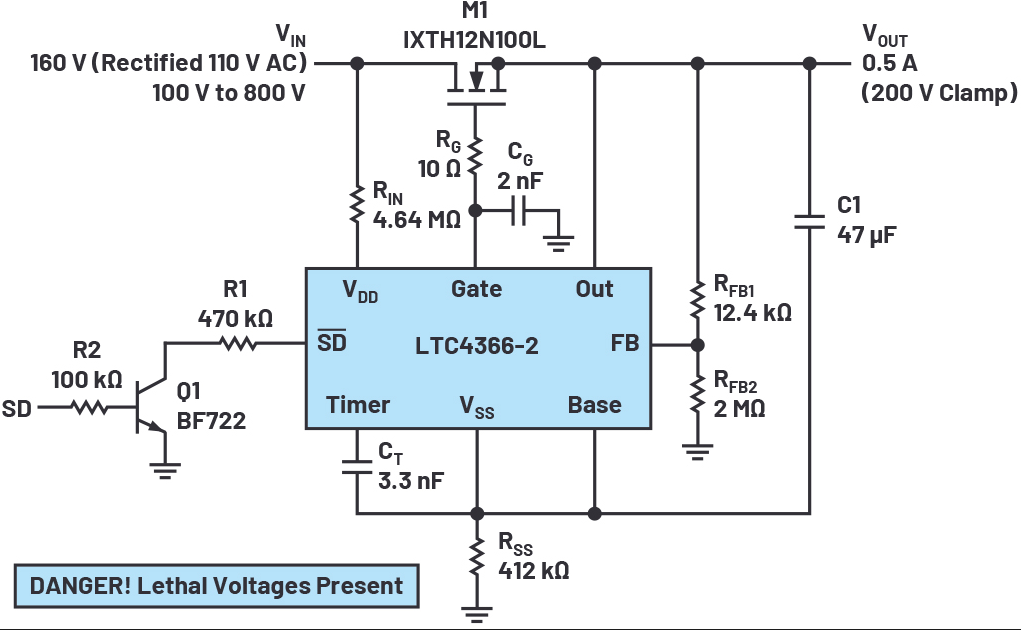
Figure 11. LTC4366 High Voltage Floating Topology
Choosing the Right Device for My Application
Surge suppressors simplify the design of protection circuits in many ways because of their inherently robust design. Data sheets already show many possible applications and can be a great help when sizing components. The hardest part may be choosing the most appropriate device. You can follow a few steps to narrow the field:
Access ADI's protection device family parametric tables.
Select the input voltage range.
Select the number of channels.
Filter functionality to narrow down the possible options.
As with all product selections, it is important to understand your system requirements before searching for the right device. Some important considerations include the expected supply voltage and voltage tolerance of downstream electronics (important when determining clamping voltages), as well as features that are important to the design.
Below are some selected parameter table examples for your reference. You can visit the website to further modify these parameter tables and add some other parameters.
High Voltage Surge Stopper Devices See Here.
Protection controllers with overvoltage disconnection function can be found here.
in conclusion
Regardless of the type of surge suppressor used, IC-based active surge suppressor designs do not require the use of complex TVS diodes or large inductors and capacitors for filtering. Therefore, the overall solution area is smaller and the volume is smaller. Compared with TVS, its output voltage clamping accuracy may be 1% to 2% higher. This prevents over-design and enables the selection of downstream components with tighter tolerances.
The portfolio of system protection devices offered by ADI enables designers to employ reliable, flexible, and compact solutions to protect downstream devices that may be exposed to severe overvoltage and overcurrent events in industrial, automotive, aerospace, and communications designs.
References
“AN-9768: Transient Suppression Devices and Principles.” Littelfuse, January 1998.
“Fuseology.” Passenger Car Solutions Catalog, Littelfuse, 2014.
Jim Kalb. “Total Fusing Time” Technical Brief, OptiFuse, January 2010.
Megaw, David. “Powering and Protecting Automotive Electronic Systems Without Switching Noise and at Up to 99.9% Efficiency.” Analog Dialogue, Volume 54, Number 1, February 2020.
Bin Wu and Zhongming Ye. “Complete Power System Design for Harsh Automotive Environments Takes up Minimal Space, Preserves Battery Power, and Has Low EMI.” Analog Dialogue, Volume 53, Number 3, August 2019.
|